Introduction
Superabsorbent hydrogels (SAHs) can absorb and retain water or aqueous fluids weighing more than 100-1000 times of their dry weight. The SAH is a lightly crosslinked hydrophilic functional polymer comprising a flexible chain structure. Because these polymers are chemically or physically crosslinked, they have some properties which can be water-swellable not water-soluble. The swelling mechanism is exactly the same as those for dissolution mechanism. Based on these excellent properties, SAH has been widely used in many specialized fields those including agriculture, horticulture, waste water treatment, drug-delivery systems, and personal disposable hygiene.1-3
The development technology and performance of SAH has been driven by growth of demands in the disposable hygiene field. Ultrahigh absorption performance of SAH has allowed the development of the ultra-thin baby diaper which uses fluff pulp.4 Also, as the absorption capacity of SAH increases, so studies on various properties of the SAH have been conducted for commercial applications. SAH has a number of factors to consider, depending on the commercial field. In particular, SAH used in the sanitary sector has the highest retention capacity, absorbency under load, ability to hold absorbed water, permeability and lowest residual monomer. Moreover, they must be non-toxic to humans and biocompatible. It is difficult for a polymer structure to meet all of these requirements simultaneously. Also, the factors are inversely related to each other. Therefore, the properties of SAH must be considered according to how they are used.5,6
To improve these performances, in this paper, I prepared an SAH nanocomposite using nanoclay. Nanocomposite structures with nanoclay can not only improve mechanical strength and absorption properties, but also reduce the production costs.7,8 Polymer/clay nanocomposites frequently exhibit excellent physical, mechanical, and absorption properties, compared to SAH without nanoclay or conventional superabsorbent composites, by the nanoscale dispersion of clay.9 After SAH nanocomposite was synthesized, post-treatment processes and surface-crosslinking were applied to the obtained hydrogel and SAH nanocomposite particle.
Experimental
Itaconic acid (IA, catalog number 97-65-4, Sigma-Aldrich Inc., China), vinylsulfonic acid sodium salt solution (VSA, catalog number 3039-83-6, Sigma-Aldrich Inc., USA), and sodium hydroxide (NaOH, catalog number 1310-73-2, Samchun Inc., Korea) were used without further purification. Tetra(ethylene glycol) diacrylate (TTEGDA, catalog number 17831-71-9, Sigma-Aldrich Inc., USA) was used as inner crosslinker. Montmorillonite (MMT, catalog number 1318-93-0, Sigma-Aldrich Inc., USA), kaolin (catalog number 1332-58-7, Sigma-Aldrich Inc., USA), bentonite (catalog number 1302-78-9, Sigma-Aldrich Inc., USA), and halloysite (catalog number 1332-58-7, Sigma-Aldrich Inc., USA) were used as inorganic clay. 1.4-butandiol (BD, catalog number 110-63-4, Sigma-Aldrich Inc., USA) was used as surface-crosslinker without further purification. Ammonium persulfate (APS, catalog number 7727-54-0, Sigma-Aldrich Inc., Japan) was used as initiator.
IA is effective for increasing the absorption capacity of SAH using hydrophilic dicarboxylic acid. Figure 1 shows synthetic procedures of SAH using IA and comonomer VSA. In this study, IA and VSA were used for the preparation of the SAH. TTEGDA with diacrylate functional group was used as inner crosslinker.1-3
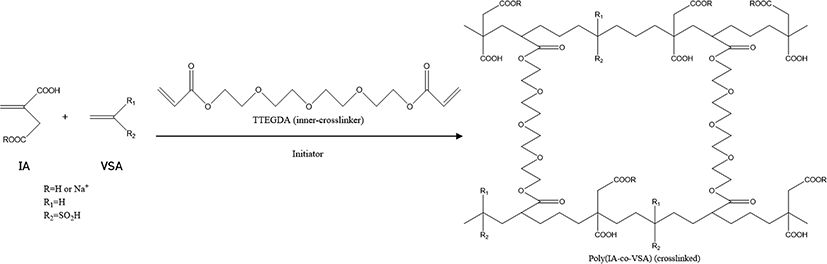
IA and VSA were subjected to 500 mL reactor which is purged with nitrogen gas and equipped with mechanical stirrer. The 40 mL of NaOH solution (54.4%) was poured into the reactor slowly with stirring 250 rpm. Then the reactor was heated to 50-70°C. Then the inner crosslinker was injected into the reactor and mixed with monomers.
In the case of superabsorbent nanocomposites, nanoclay or silver nitrate solution was added into the medium. Under the constant reaction temperature, an initiator was input into the reactor. The polymerization was stopped when further stirring becomes impossible due to the high viscosity of the reaction medium. The products dried in an oven at 120°C for 30 min. I ground and classified the SAH in a particle size ranges of 300-500 μm.
After the grinding and classification step, the surface-crosslinking of the products was conducted. Figure 2 shows summary of experimental process to prepare SAH nanocomposite. Also Table 1 shows the procedure and method for each post-treatment process and surface-crosslinking process.
Centrifuge retention capacity (CRC) is a measurement method to know how much moisture is retained by dehydrating the swollen SAHs with a centrifuge. This test method refers to EDANA WSP 241.2. Distilled water was added to the 200 ml beaker and NaCl were introduced to make 0.9 wt% NaCl aqueous solution. Weigh accurately 0.1 g of SAH particle using the heritage and carefully put into a 100 mesh wire netting tea bag. Take out the tea bag from the solution after 30 minutes, then wipe the water off the tea bag. Centrifuge for 3 minutes at 300 G of gravity to dehydrate. Measure the mass of swelling SAH carefully after centrifugation. The CRC was obtained using Eq. (1), where W1 is the weight of the swollen SAH and W0 is the weight of the dried SAH.
Absorbency under load (AUL) is a measurement method to know how much moisture is absorbed under the pressure. This test method refers to EDANA WSP 242.2. Place the ceramic filter plate (d = 80 mm, h = 5 mm) on the petri dish, cut the filter paper slightly larger than the plate, and place in on the ceramic filter plate. Place the tea bag on the filter paper and the glass cylinder (d = 60 mm, h = 50 mm) in the center of the filter paper. Spray 0.9 g of dried SAH on the inside of the cylinder evenly, then pressurize the cylinder with a piston (0.3 psi, h = 50 mm). Take care not to touch the 0.9 wt% NaCl aqueous solution directly on the ceramic filter plate and pour into the petri dish. Weigh the swollen SAH after 60 minutes. The AUL was obtained using Eq. (2), where W1 is the weight of the swollen SAH and W0 is the weight of the dried SAH.
Water permeability is a measurement method to know how much moisture passing through the swelled gel layer. 0.5 g of SAH is added to the cylinder and allowed to swell for 30 minutes on the 0.9 wt% NaCl aqueous solution. A pressure device with 0.3 psi is placed over the swollen SAH. Open the cock at the bottom of the cylinder and measure the time it takes for 20 ml of 0.9 wt% NaCl aqueous solution to be received.
ATR-FTIR (Nicolet, NEXUS) was used for analyzing the structure of SAH. XRD (X Pert-Pro MRD XL, Malvern Panalytical) was used for observing X-ray patterns of SAH nanocomposite according to the nanoclay input content. SEM (JSM-6390/LV, JEOL) was used for observing surface morphology microstructure of SAH according to quenching process.
Results and Discussion
Figure 3 shows the spectrum of ATR-FTIR for the prepared SAH. The broad absorption band at 3200-3600 cm−1 can be attributed to hydroxyl groups in IA. The band observed at 1714 cm−1 can be attributed to C=O stretching in carboxylate functional groups in IA and inner crosslinker. Absorption peaks at 1182 and 1047 cm−1 are appeared due to the sulfonyl group. The characteristic band at 1550 cm−1 is due to the C=O asymmetric stretching in carboxylate anion that is reconfirmed by another peak at 1449 cm−1 which is related to the symmetric stretching mode of the carboxylate groups.
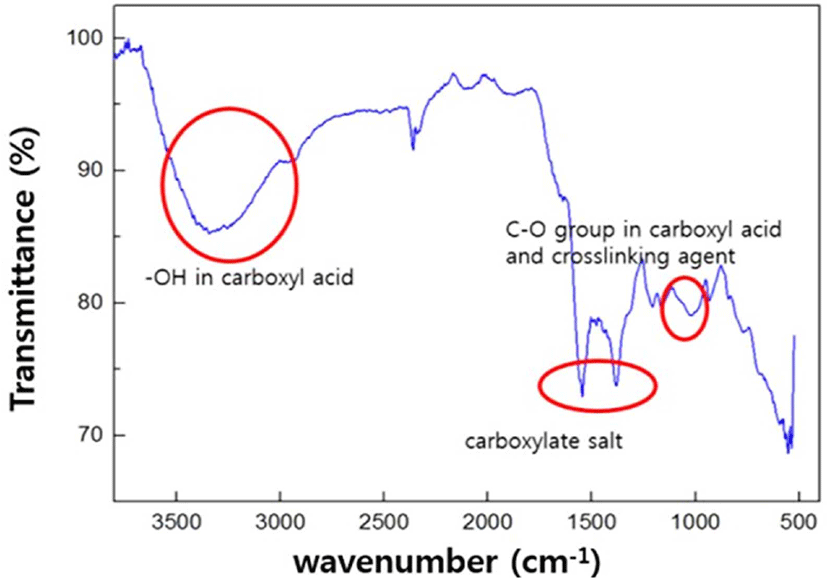
Clay nanocomposites exhibit markedly improved mechanical, thermal, optical, and physical chemical properties when compared with the pure polymer or conventional (microscale) composites as firstly demonstrated by Kojima and coworkers for nylon-clay nanocomposites. Improvements can include, for example, increased modulus, strength and heat resistance, decreased gas permeability, and flammability.9,10
Depending on the nature of the components used (layered silicate, organic cation and polymer matrix) and the method of preparation, three main types of composites may be obtained when a layered clay is associated with a polymer (see Figure 4). When the polymer is unable to intercalate between the silicates sheet, a phase separated composite (see Figure 4a) is obtained, whose properties stay in the same range as traditional micro composites. Beyond this classical family of composites, two types of nanocomposites can be recovered. Intercalated structure (see Figure 4b) in which a single (and sometimes more than one) extended polymer chain is intercalated between the silicate layers resulting in a well ordered multilayer morphology built up with alternating polymeric and inorganic layers. When the silicate layers are completely and uniformly dispersed in a continuous polymer matrix, an exfoliated or delaminated structure is obtained (see Figure 4c).9
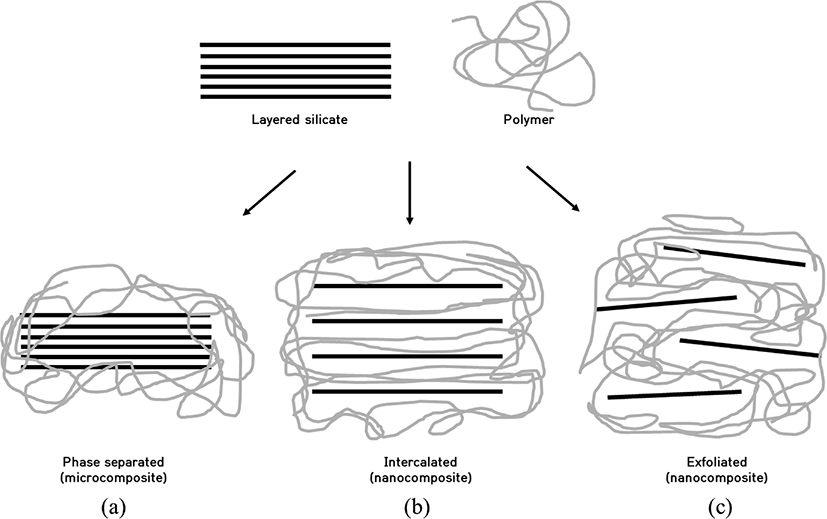
The purpose of this study is to determine the improved properties of SAH clay nanocomposite and to improve their absorption performance and mechanical properties. According to the type and content of nanoclay in the SAH network, the dispersibility of the clay and improved characteristics of the SAH were investigated. Table 2 shows the types and general formula of clay minerals used.
Clay | General formula |
---|---|
Kaolin | Al2O3·2SiO2·2H2O |
Halloysite | Al2Si2O5·(OH)4·2H2O |
Montmorillonite | (AI, Mg)2Si4O10·(OH)2·4H2O |
Bentonite | Al2O3·4SiO2·H2O |
Figure 5 shows XRD patterns of pure nanoclay, the SAH without nanoclay, and SAH clay nanocomposite according to the type and content of input nanoclay. XRD pattern of pure nanoclay shows a strong peak. After copolymerization, this peak of SAH clay nanocomposite has almost disappeared. When compared with the SAHs without nanoclay, up to 2 phr, no specific peak is seen by adding clay. As far as exfoliated structure is concerned, no more diffraction peaks are visible in XRD diffractograms either because of too much large spacing between the layers exists (i.e. exceeding 8 nm in the case of ordered exfoliated structure) or because of little ordering. Results obtained indicate that nanoclay powder has been finely dispersed in SAHs matrix, and the copolymerization reaction is performed on the surface of nanoclay powder.13,14 On the other hand, when the clay was above 10 phr, specific peaks appeared at 2θ similar to pure clay. It seems that the clay is not well dispersed in the copolymer network.
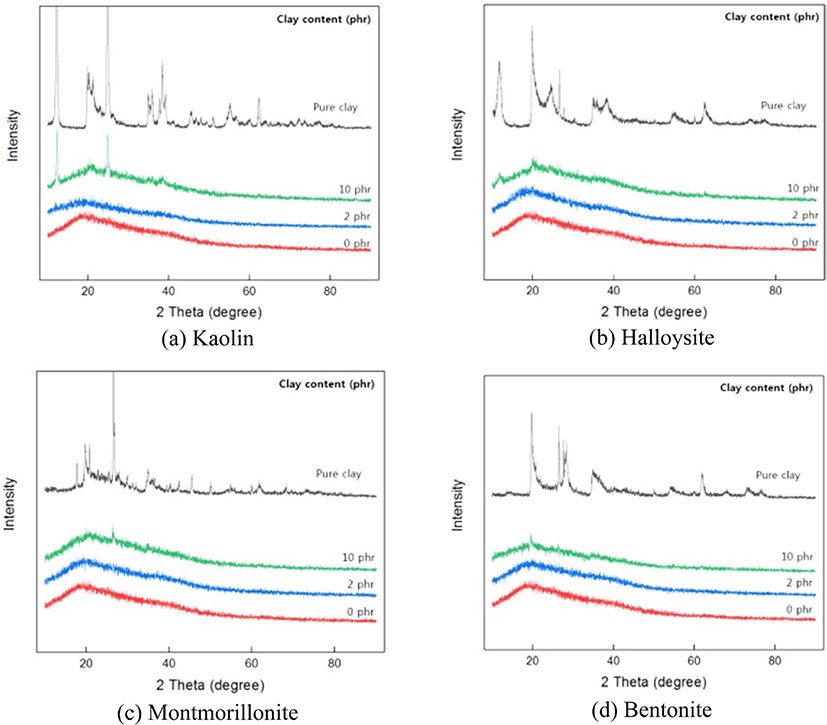
Figure 6 (a and b) and 14 show absorption properties of SAH clay nanocomposite according to the type and content of nanoclay. When compared with SAH without nanoclay, SAH using nanoclay showed a higher CRC and AUL (see Figure 6a). Also, under the critical point, as the content of nanoclay in the nanocomposites increased, the absorption performance of SAH increased (see Figure 6b). This seems to be attributed to the increase of the intermolecular interaction such as hydrogen bonding caused by the side chains of nanoclay and water. Nanoclay made the crosslinking density of SAH high due to the occurrence of the additional physical crosslinking. Therefore, the addition of nanoclay to SAH network increased CRC and AUL.
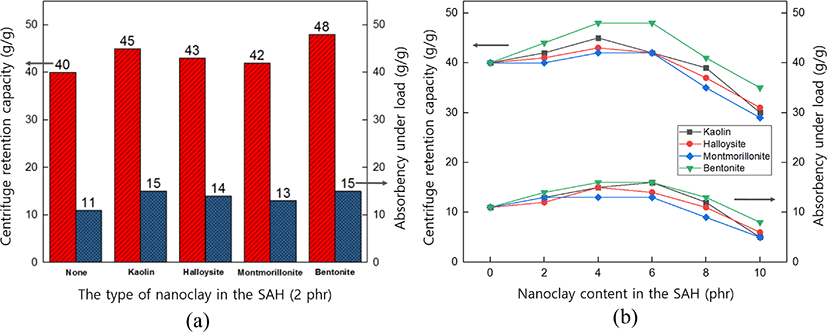
On the other hand, above the critical point, as the content of nanoclay increases, the cross-linking density becomes high and the space to absorb water decreases. Also, when high excessive amount of nanoclay added, SAH can dissolve in water because it interfered the crosslinking reaction. Therefore, to increase the absorption capacity of SAH, it is important to add an appropriate amount of nanoclay.
The quenching process is to soak SAH in the organic solvent immediately after polymerization. At this time, acetone, ethanol, and methanol, which are not reactive with SAH network and easy to evaporate from water, were used as the organic solvent. When the water in SAH network is replaced with a cold organic solvent, the polymerization reaction is terminated and porosity is formed.11Figure 7 (a and b) is SEM images of SAH network according to quenching process. As the quenching process was applied, the porosity of SAH network was confirmed.
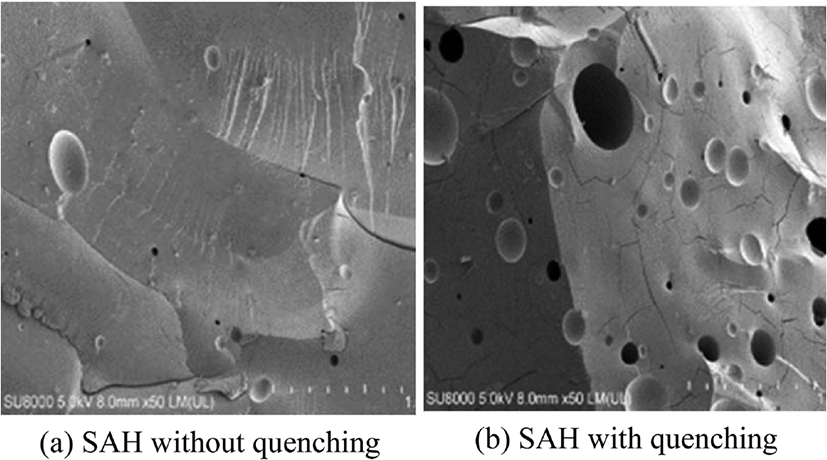
The aging process leads to an additional reaction of residual monomers of SAH. Theoretically, holding the reaction temperature after polymerization in gel is known to increase the conversion of monomers. First, the polymerized SAH is removed from the reactor and deposited in a thermo-hygrostat at a constant temperature.3
Table 3 shows the residual monomer content of SAH according to the post-treatment process by HPLC. SAH with post-treatment process showed a significant decrease in residual monomer content. The quenching process showed the lowest residual monomer content due to the cleaning action. On the other hand, compared to the quenching process, the aging process reduced the residual monomer by increasing the monomer conversion ratio.12
Post-treatment process | None | Aging process | Quenching process |
---|---|---|---|
Residual monomer (mg/L) | 128.00 | 3.32 | 0.81 |
Table 4 also shows the change of absorption properties of the SAH by aging process. CRC and AUL of SAH increased when the aging process was applied. This seems to be an increase in the amount of water that can be absorbed by the expansion of SAH network. Therefore, it is believed that if the quenching process is introduced after the aging process, the conversion ratio and absorption properties of SAH will increase and the residual monomer will decrease significantly.
Post-treatment process | CRC (g/g) | AUL (g/g) |
---|---|---|
None | 40 | 12 |
Aging process | 45 | 15 |
The surface-crosslinking process enhances the gel strength by forming a core-shell structure in SAH. The surface on SAH was crosslinked by chemical reaction using carboxylic acid functional group on SAH surface. As the hydrophilic functional group on SAH surface is crosslinked, the water permeability increased. Also, with the surface-crosslinking density of the surface, gel strength increased.
Figure 8 shows the synthetic mechanism of surface-crosslinking of SAH using 1,4-butanediol (BD) as a surface crosslinker. The carboxylic acid group on SAH surface reacted with the dialcohol of BD to form an ester bond. With the esterification reaction, surface of SAH were crosslinked and formed core-shell structure in SAH.
Table 5 shows the absorption properties and water permeability according to the surface-crosslinking. The increase in gel strength after surface-crosslinking of SAH significantly improved AUL of SAH. On the other hand, a decrease in CRC of SAH was observed, because the absorbed capacity of SAH network decreased due to the increased crosslink density of SAH. It was confirmed that when the surface of SAH was crosslinked, water permeability of SAH was improved.
CRC (g/g) | AUL (g/g) | Water permeability (s) | |
---|---|---|---|
None* | 40 | 12 | 300 |
Surface-crosslinking** | 35 | 20 | 60 |
Conclusions
Poly(IA-co-VSA) was prepared as the SAH using radical copolymerization. SAH nanocomposites were synthesized to improve absorption performance and functionality. Optimal dispersion conditions of organic/inorganic nanoclay in the composites were confirmed by XRD. The nanoclay improved CRC and AUL of the composites by increasing crosslink density and hydrophilic functional groups. I found porous structure of SAH using the quenching process by SEM. The conversion of the residual monomers, analyzed by HPLC, was improved by the aging process. Also, surface-crosslinking process improved AUL and water permeability by forming a core-shell structure in SAH network. In addition, surface-crosslinking process improved AUL and water permeability by forming a core-shell structure in SAH network.