Introduction
가교는 고분자 사슬간의 물리적 혹은 화학적 상호작용에 의 한 연결이다. 물리적 가교는 사슬의 엉킴이나 사슬간의 이온 결합과 같은 상호작용에 의한 것이고, 화학적 가교는 분자 사 슬간의 화학 결합에 의한 것이다. 고무를 가교시킬 경우 고무 사슬간에 3차원 망상 구조가 이루어진다 (Figure 1). 망상 구조 를 이룰 경우 고무 사슬을 신장시켜도 본래의 상태로 돌아가 는 탄성을 갖는다. 가교된 고무는 우수한 물성과 화학적 특성 을 갖기 때문에 가교되지 않은 고무보다 제품으로 활용하기 에 적절하다. 이 때문에 고무를 가교하는 과정이 요구된다.
20세기 초 황에 의한 고무의 가교가 발견되면서 아민계 촉 진제와 같은 다양한 물질을 사용하는 가교 기술이 발전하였 다.1 황에 의한 가교뿐만 아니라 과산화물이나 레진 등에 의 한 가교가 개발되었다.2 더불어 촉진제나 충진제 등 다양한 물 질을 첨가하였는데, 이에 따라 가교밀도가 달라질 수 있다. Figure 2 에 가교밀도에 따른 물성의 변화를 나타내었다.3 가 교밀도가 증가할 수록 경도와 모듈러스는 증가하고 신율은 감 소한다. 인장강도는 일정수준 이상 증가하다 감소하는 경향 을 보인다. 인장강도는 신장된 고무사슬의 배향에 의해 결정 화가 일어난 이후에 사슬이 끊어지면서 결정된다.3,4 가교밀도 가 일정수준 이상 증가하면 가교점이 많아져 고무사슬의 배 향이 제한되기 때문에 인장강도가 감소한다.3-5 고무에 황을 첨가하여 가교밀도가 물성에 미치는 영향을 확인하는 연구가 다수 진행되었다.6-10 과산화물에 의한 가교는 고분자의 탄소-탄소 화학결합에 의해 이루어지기 때문에 황에 의한 가교보 다 가교길이가 짧다. 따라서 가교밀도가 동일할 경우, 황에 의 한 가교물은 과산화물에 의한 가교물보다 상대적으로 낮은 유 리전이온도를 가진다.11 황의 다황가교와 고리형 황화물 구조 가 유리전이온도에서 사슬의 배향에 영향을 미친다.11
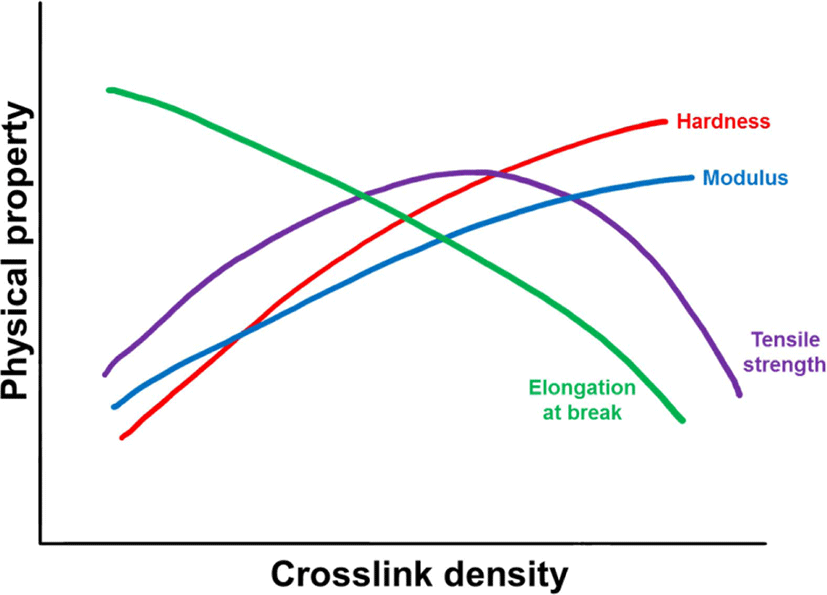
황에 의한 가교는 황과 가교촉진제, 산화아연(ZnO), 스테아 린산(stearic acid) 를 첨가하여 고무사슬에 단황 (~C-S-C~), 이 황(~C-S2-C~), 혹은 다황 (~C-Sn-C~, n ≥ 3)의 결합을 이루게 하는 가교이다. 황이나 가황촉진제의 황 결합은 열에 의해 잘 분해되어 라디칼을 생성한다. 결합이 깨진 황은 고무사슬에 황 결합을 도입한다.2 황 가교를 속도를 조절하기 위해 가해 지는 가교촉진제에는 guanidine, thiazole, sulfenamide, dithiocarbamate, thiuram, thiourea 계 등이 있으며, 그 종류에 따라 가교 특성에 미치는 영향이 다르게 나타난다. 황과 가교촉진 제의 비율을 다르게 하였을 경우 황 가교의 종류가 달라지기 때문에 가교촉진제 /황(A/S) 비율에 따라 conventional (< 0.7), semi-EV (0.7-2.5), EV (> 2.5) 가교시스템으로 나눌 수 있다.2 일반적으로 A/S 비가 증가함에 따라 단황 가교는 증가하고 다 황 가교는 감소한다. 단황과 다황 가교의 비율은 고무의 물성 에 큰 영향을 주기 때문에 이들을 분석하는 연구가 진행되고 있다.12-18
과산화물은 산소-산소(O-O) 결합이 열이나 자외선에 의해 분해되어 라디칼을 이루고, 사슬 내 수소를 제거하여 사슬간 탄소-탄소 결합을 이루게 한다. 포화 탄화수소로 이루어져 있 는 고분자에서도 가교가 잘 일어나기 때문에 PE나 실리콘 고 무, EPR의 가교에도 이용된다.2 탄소-탄소 간의 결합이 이루 어지기 때문에 가황 가교보다 상대적으로 열노화 저항성이 좋 지만, 배합물 내 다른 성분과 결합하여 가교효율이 낮아질 수 있는 제약이 있다. 이외에도 알킬페놀-포름알데히드 레졸 수 지를 가해 Diels-Alder 반응을 이용한 가교,2 실리콘 고무의 백 금촉매를 이용한 가교,19 아미노산, 수분을 이용한 가교2 등이 있다. 전자 빔을 이용해 라디칼을 생성하여 탄소-탄소 결합을 만드는 가교, 고무에 자외선 흡수제를 가하여 자외선을 조사 하여 가교를 시키는 방법 또한 이용되고 있다.2Table 1에주 요 가교 시스템의 종류와 응용 고무 종류를 정리하였다.
가교제의 종류와 함량 그리고 가교조건에 따라 가교밀도가 달라져 물성이 달라지기 때문에 가교밀도를 분석하는 것이 중 요하다. 본 논문에서는 고무 가교물의 가교밀도를 구하는 방 법을 서술하였다. 가교밀도를 분석하는 방법은 고무와 상호 작용이 좋은 용매(good solvent)에 담가 팽윤시켜 무게를 측 정하는 팽윤법,37,38 NMR을 이용한 방법,20 가교 중 운동상수 분석 방법,39 DSC를 이용한 방법21 등이 개발되었다. 그 중에 서도 가장 널리 이용되는 팽윤법과 NMR을 이용하여 가교밀 도를 분석하는 방법을 서술하였다.
Measurement of Crosslink Density Using Swelling Method
가교된 고무는 좋은 용매에 담그면, 용매 분자가 고무사슬 내로침투 한다. 가교밀도가 높을수록 가교된 고무 사슬 사이 로 용매 분자가 침투할 수 있는 양이 적어지기 때문에 상대 적으로 덜 팽윤된다. 이러한 현상을 이용하여 팽윤비(Q)를구 하고, 이를 역수로 하였을 경우 겉보기 가교밀도(1/Q)로 사용 한다. 겉보기 가교밀도를 구하는 식은 다음과 같다.40-43
여기서 Ws는 팽윤된 가교물의 무게, Wu는 팽윤되지 않은 가 교물의 무게, Wsol 은 팽윤 용매의 무게이다. 팽윤되지 않은 가 교물은 잔류 유기물을 제거한 것을 말한다. 겉보기 가교밀도 를 정량적으로 나타내기 위하여 많은 연구가 진행되어왔다. 식 (2)의 Flory-Rehner 식을 이용하면 가교밀도(Xc)를 구할 수 있다.44-46
여기서 v2는 고분자의 부피분율, χ는 고분자-용매간 상호작용 상수, V1는 용매의 몰부피(cm3/mol)이다. 고분자의 부피분율 은 앞서 서술한 팽윤비를 이용하여 식 (3)으로부터 구할 수 있다.
여기서 Wr은 팽윤되지 않은 가교물 중 고무의 무게, Wsol은 팽윤용매의 무게, ρr은 고무의 밀도, ρsol은 팽윤용매의 밀도 이다. 팽윤비에서 고무와 팽윤용매의 밀도를 이용하면 Flory-Rehner식에서 고분자의 부피 분율을 구할 수 있다. Flory-Rehner 식은 격자 용액 이론을 기반으로 하기 때문에 몰분율 이나 무게분율이 아닌 부피분율을 이용한다.
Interaction Parameter
Flory-Rehner 식에서의 상호작용 상수(interaction parameter, χ)는 고무와 팽윤용매 간의 상호작용의 차이에 의한 팽윤 정 도의 차이를 보정하기 위해 필요한 값이다. 고무와 용매간의 상호작용에 따라 고무의 팽윤도가 달라지기 때문에 올바른 상 호작용 상수를 이용하는 것이 매우 중요하다. Table 2에 주요 고분자와 용매간의 상호작용 상수를 정리하였다.47-65χ 값을 구하는 방법으로는 용해도상수를 이용하는 방법과 역기체크 로마토그래피(inverse gas chromatography)를 이용하는 방법 등이 있다.
Solvent | Polymer | Reference | |||
---|---|---|---|---|---|
NR | BR | SBR | EPDM | ||
n-Heptane | 0.5 | - | - | 0.417-0.430 | 47, 48 |
n-Decane | 0.43 | 0.463 | - | - | 49, 50 |
Cyclohexane | 0.310 | 0.426 | 0.489 | 0.369-0.387 | 48, 51, 52 |
m-Xylene | 0.4106 | - | 0.3908 | 0.406-0.419 0.477-0.481 |
48, 53, 54 |
Toluene | 0.393 | 0.34, 0.47 | 0.446 | 0.49 | 48, 55, 56, 57, 58, 59 |
Benzene | 0.451 | 0.21, 0.265, 0.325, 0.36 | 0.343 | 0.550-0.561 | 48, 51, 60, 61, 62, 63, 64 |
THF | 0.446 | - | - | 0.503-0.503 | 48, 65 |
χ 값은 엔트로피와 엔탈피의 항으로 나눌 수 있으며, 엔탈 피 기여를 χH 로엔트로피 기여를 χS 라고하였을 때, χ는 식 (4)로 표현할 수 있다.46
여기서 δS 와 δP 는 각각 용매와 고분자의 Hildebrand 용해도 상수(MPa1/2) 이다. 이에 따라 식 (4)를 이용하여 χ 값을 도출 하기 위해서는 고무와 팽윤용매의 용해도상수가 필요하다. 일반적으로 용해도상수가 유사한 물질일수록 상호작용이 좋 다. 그룹기여방법(group contribution method)를 이용하여 Hildebrand와 Hansen에 의해 결정된 팽윤용매의 용해도상수 를 Table 3에 정리하였다.66-69 용해도상수의 개념을 최초로 정 의한 Hildebrand66는 액체상에서 분자들이 응집 되어있는 상 태를 유지하기 위한 힘을응집에너지(cohesive energy)를이 용하였다. 응집에너지와 간의 관계는 식 (5)와 같다.
Materials | Solubility parameter (MPa1/2) | Method | References |
---|---|---|---|
n-Octane | 15.3, 15.5 | GCMa | 70 |
Cyclohexane | 16.0, 16.80 | GCMa | 70 |
Toluene | 18.2, 18.16 | GCMa | 70 |
m-Xylene | 18.0, 18.72 | GCMa | 70 |
Chloroform | 19.0, 18.95 | GCMa | 70 |
THF | 19.4, 19.46 | GCMa | 70 |
Methanol | 29.6, 29.61 | GCMa | 70 |
Ethanol | 26.50, 26.52 | GCMa | 70 |
Isopropyl alcohol | 23.5, 23.58 | GCMa | 70 |
NR | 17.0 | GCMa | 70 |
BR | 17.0 | GCMa | 70 |
PE | 17.0 | GCMa | 70 |
PP | 16.5, 14.74 | GCMa, IGC | 71, 72 |
PIP | 16.34 | IGC | 71 |
EPR | 14.74 | IGC | 71 |
PDMS | 14.1-13.6 | IGC | 72 |
PS | 18.5, 18.99, 18.73 | GCMa, μDSC, [η]b | 73 |
PVA | 20, 20.73 | GCMa, IGC | 70, 74 |
위 식에서 δS 는 용해도상수, ΔEν는응집에너지, Vm은 액체 상에서 물질의 몰부피, ΔUvap 는 액체상에서 이상기체상로 휘 발되기위한 에너지, ΔHvap는 기화엔탈피, R 은 기체상수이 다. 용매분자간에 작용하는 반데르발스 힘을 기화엔탈피를 통 해 측정하여 이를 용해도상수에 도입한다. 하지만 이러한 방 법은 용매분자간에 반데르발스 힘만이 작용한다는 것을 가정 하였기 때문에 분산력, 쌍극자 상호작용, 수소결합이 작용하 는 극성용매에는 적용이 불가하다는 한계가 있다. 이를 극복 하기 위하여 Hansen67은 다음 식으로 분산력, 쌍극자 상호 작용, 수소결합이 포함된 식으로 확장하였다.
여기서 δS,HSP는 Hansen 용해도 상수, δD는 분산력, δP는 쌍 극자 상호작용, δH은 수소결합에 대한 항이다. 위 세 가지 요 소를 종합하여 나타낼 경우 극성용매까지 적용할 수 있다. 이 러한 방법은 휘발성을 가지는 물질에 국한된 방법이기 때문 에 고분자의 용해도상수를 결정하기엔 제한이 있다. 고분자 의 용해도상수는 미세시차주사열량법(micro differential scanning calorimetry, μDSC), 고유점도법(intrinsic viscosity measurement), 그리고 자외선-가시선 분광법(UV-Visible spectroscopy) 을 이용하여 구할 수 있다(Table 3).71-74
저분자량 물질은 DSC를 이용하여 휘발할 때의 엔탈피값 을 구하여 식 (5)에 대입하여 용해도상수를 구할 수 있지만, 고분자 물질은 휘발되기 전에 열분해가 되어 휘발에 의한 엔 탈피 값을 측정할 수 없다. 이러한 문제를 해결하기 위해 Carvalho48는 μDSC를 이용하였다. μDSC를 이용할 경우 DSC 보다 더 높은 분자량 물질의 용해도상수를 측정할 수 있다. μDSC 내 두 부위로 나뉜 측정부에서 고분자에 용매를 두번 으로 나눠 첨가하여 용매의 용해도 엔탈피를 측정한다. 첫번째 첨가 시에는 고분자의 용해가 일어나는 온도의 변화를, 두 번째 첨가 시에는 용매가 첨가되었을 때 일어나는 온도변화 만을 측정한다. 이후 첫 번째 용매가 첨가된 엔탈피에서 두번 째 용매가 첨가된 엔탈피의 차이가 고분자 용해 엔탈피이다. 용매의 조성을 다르게 할 경우 용해 엔탈피가 가장 클 때, 그 용매의 혼합물의 용해도 상수가 고분자의 용해도 상수라고 할 수 있다.
고무와 용매의 상호작용이 강할수록 고분자 사슬이 잘 풀 어져 고유점도가 증가한다. 이에 따라 고분자의 용해도 상수 는 고유점도가 가장 높은 용매 조성과 유사하다. 점도 측정계 를 이용하여 다양한 용매 비율을 가진 고분자 용액의 고유점 도를 측정한다.48 일반적으로 Ostwald 점도계를 이용하여 일 정 온도에서 고분자 용액의 흐름시간을 측정하며 고유점도를 구한다. 이후 두 가지 용매를 혼합하여 다음 식으로부터 용해 도 상수를 구한다.
여기서 δm 은 고분자의 용해도상수, δ1과 δ2 는 서로 다른 순 수한 용매의 고분자상수, ϕ1과 ϕ2는 혼합 용매에서 각 용매의 부피비이다. 고유점도가 가장 높은 용매의 부피비에서 식 (7) 로부터 고분자의 용해도상수를 구할 수 있다.
고분자 용액에서 고분자와 용매 간의 상호작용이 강하면 용 액에서 더 많은 분자가 빛을 흡수할 수 있는 것을 이용하여 용해도상수를 구할 수 있다.48 고분자가 좋은 용매에 녹으면 고분자 사슬이 용액 내에서 잘 풀어진 상태로 분포한다. 용매 가 고분자와 잘 상호작용 할수록 고분자 사슬은 더 잘 풀어 진 상태로 분포하기 때문에 자외선-가시선 분광기로 측정하 였을 경우 투과되는 광량이 낮다. 이에 따라 고분자의 용해도 상수와 가장 유사한 용매의 부피 조성에서 강한 흡광도를 보 인다. 이러한 용매의 부피 조성으로부터 식 (7)에 따라 고분 자의 용해도상수를 구한다. 반면 위방법을 이용할 경우고분 자가 용액에 충분히 녹지 않아 용액의 혼탁도가 높으면 흡광 도가 높게 측정될 수 있기 때문에 적절한 용매의 선정이 중 요하다.
IGC는 고분자와 용매가 상호작용을 잘 할수록 늦게 용리하 여 머무름 부피가 증가하는 원리를 이용한다.48 컬럼 고정상에 대상 고분자를 도포하고 기체 상태의 용매를 용리시킨다. 컬 럼 입구와 출구의 압력강하를 보정하기 위한 James-Martin 압 력 보정인자(j), 운반기체의 흐름속도 보정인자 (F), 용리 시간 과 컬럼 내에 포함된 고분자의 양 (Ws)을 식 (8) 에 대입하여 머 무름 부피 (Vg)를 구할 수 있다. 식 (8) 에서 tr 과 trm은 각각 주 입한 용매 시료와마커 (marker) 의 머무름 시간이다.
머무름 부피를 식 (9) 에 대입하여 무한 희박 활동도상수 ln(Ω∞)로 계산한 후 상호작용상수를 구할 수 있다.
식 (9) 에서 R은 기체상수, T는 IGC 측정 온도, M1은 용매 의 분자량, p0은 측정 온도에서의 용매의 증기압, B11은제 2 차 비리얼상수, V1은 용매의 몰 부피이다. 식 (10) 에서 V1과 V2 는 각각 용매와 고분자의 몰부피이고, r1과 r2는 각각 용매와 고분자의 밀도이다. 용매의 몰부피는 고분자의 몰부피보다 현 저하게 낮기 때문에 V1/V2는 0에가깝다. 이러한 방법을 통해 고분자나 용매의 용해도 상수의 계산 없이 고분자와 용매의 상호작용 상수를 구할 수 있다.
Measurement of Crosslink Density Using NMR
자기장 하에 놓인 화합물의 짝을 이루지 않은 원자 핵의스 핀은바닥 상태와 들뜬 상태로 분리되며, 이들의 에너지 차이 는 라디오파(RF)에해당된다. 공명 RF 를 조사하면 흡수가 일 어난다(Figure 3). 들뜬 상태의 스핀이 원래 상태로 돌아가는 것을 이완(relaxation)이라고 하는데, 스핀-격자 이완(spin-lattice relaxation, T1)과스핀-스핀 이완(spin-spin relaxation, T2)이 있다. 스핀-격자 이완은스핀과 주변 격자들의 열적 평 형이 이루어지면서 에너지가 교환되는 것에 기인한다. 스핀 이 높은 에너지 상태에서 낮은 에너지 상태로 갈 경우 RF 에 너지는 주변 격자들로 이동하면서 스핀 이완이 일어난다. 스핀-스핀 이완은 같은 종류인 두 개의 이웃한 핵이서로 다 른 자기양자수를 갖지만 동일한 세차운동 속도를 갖고 있을 때, 양자 상태의 상호교환에 기인한다 (Figure 4). 이러한 스핀-스핀 이완을 이용하여 가교밀도를 구하는 방법이 연구되었 다.71-80Table 4에 고무 종류와 가교 시스템이 다른 경우에 NMR을 이용하여 가교밀도를 측정한 문헌을 정리하였다. 가 교된 고무의 분자구조에 따른 T2시간의 변화를 Figure 5 에나 타내었다. 가교된 사슬은 가교되지 않은 사슬보다 T2시간이 빠르기 때문에 이를 이용하여 가교밀도를 구할 수 있다.
Method | Polymer | Filler | Cure system | References |
---|---|---|---|---|
Solid 1H-NMR | PE/PP | - | Peroxide | 72 |
EPDM | - | Peroxide | 73 | |
NR | - | Sulfur | 74 | |
BR | Carbon black | Sulfur | 75 | |
EPDM/PP | - | Peroxide | 76 | |
NR | Carbon black | Sulfur | 77 | |
NR | Carbon black | Sulfur | 78 | |
Solid 13C-NMR | NR | - | Sulfur | 74 |
NR | Carbon black | Sulfur | 78 | |
Liquid 1H-NMR | NR | Carbon black | Sulfur | 79 |
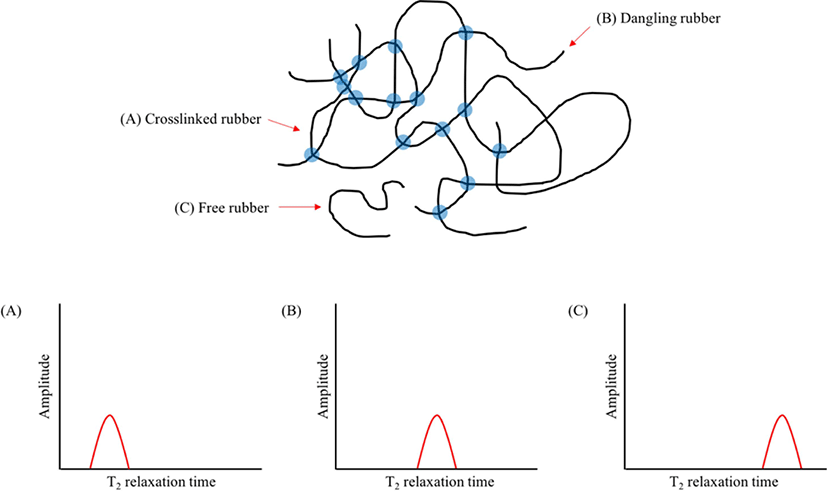
고체NMR을 이용하여 가교물을 분석할 경우, 온도를 증가 시키며 가교물의 T2시간을 측정할 때, T2시간이 일정하게 유 지되는 값 (high temperature plateau T2 relaxation, T2P)을 통해 가교 세그먼트의 정량적 분석이 가능하다.72 가교물의 Tg로부 터 100~150 K 이상에서 T2 시간을 측정하여 화학적, 물리적 결합의 평균 위치를 계산할 수있다. T2P를 이용하여 가교밀 도를 구하는 식은 다음과 같다.
여기서, Z는 가교점의 개수를 뜻하고, a 는 사슬의 주축에서 가장 가까운 핵스핀에 대한 세그먼트 축과 내부핵벡터 사이 의 각에의존하는 이론적인 계수이다. 지방족 양성자를 가지 는 고분자의 경우 a값은 6.2 에 근사한다. T2rl 값은 Tg 이하에 서 고무사슬에 의해 이루어진 단단한 격자 (rigid lattice) 내수 소-수소 간 상호교환에 기인한 T2 시간이다. 이로부터 네트워 크 내 가교점의 몰질량 (Mw)은 식 (12) 과 같이 나타낸다. 앞서 언급한 Z값과 공중합체의 기본사슬의 수평균몰질량(Mu), n은 기본 사슬 내 탄소간의 결합 수, C∞은시편 내 사슬의 통계 적 개수이다.
들뜬 스핀이이완하면서 방출하는 전자기복사선이 자유 유 도감쇠(free induction decay, FID) 신호이다. 이러한 FID 신 호를 이용하여 황 가교의 길이를 측정할 수 있는 방법 또한 고안되었다.71 황에 의한 가교결합은 단황, 이황, 다황 결합으 로 다양하게 구성되어있는데, 13C-NMR을 이용하여 가교결합 의 종류와 평균적인 황의길이를 분석할 수있다. 황 가교의 결합 길이는 다음 식으로 나타낸다
여기서, Lc는 가황고무 내 황 가교 결합의 길이, MS는 가황 고무 내 황 원자의 몰수, AR은 가황 고무의 FID 증폭 값, AST 는 표준물질의 FID 증폭 값, nC,ST 는 표준물질의 특징 피크에 해당하는 원자의 표준 물질의 분자 내 개수, MST는 표준물질 의 몰수이다. 톨루엔을 표준 물질로 이용하여 천연고무의 chemical shift 를 통해고무 내 cavity size 를 측정하여 위 방 법의 재현성을 나타낸다.71
NMR을 이용하여 얻은 가교밀도는 팽윤법을 이용하여 얻 은 가교밀도와 다소 차이가 있을 수 있다. Litvinov 는 T2P값을
이용하여 가교밀도를 나타낼 경우, a값과 C∞의 값이 정확하지 않기 때문에 15~25% 의 오차가 존재할 수 있음을 보고하 였다.72 또한 Dibbanti 는 팽윤법보다 고체 NMR로 측정하였을 때 가교밀도가 상대적으로 크게 나타날 수 있음을 보고하였 다.80 이는 팽윤된 고무의 상태보다 팽윤되지 않은 고무의 상 태에서 얽힘이더 심하고, 고체NMR에서 이러한 얽힘까지고 려하여 T2 값을 나타내기 때문이다.
Measurement of Mono-, Di-, and Polysulfidic Crosslink Densities
가황 과정에서는 고무사슬 간에 단황, 이황, 다황 결합이 이 루어져 가교를 이룬다. 황 결합들의 비율은 고무 소재의 물성 에 영향을 주기 때문에 이를 분석하는 연구가 이루어졌다.81-89 이황과 다황 가교 결합을 끊는 과정과 다황 결합만을 끊는 과 정을 통해서 세 가지 황 가교 결합의 비율을 알 수 있다. Thiol 과 amine 화합물을 이용하여 이황과 다황 가교 결합을 끊는 방법이 연구되었다.85-89 Propane-2-thiol 과 piperidine 을 이용하 여 다황 가교 결합을, hexanethiol 과 piperidine 을 이용하여 이 황과 다황 가교 결합을 끊는 방법이 먼저 연구되었다.85-86 Kiroski는 앞서 이용된 용매인 n-heptane 대신 toluene 을 이용 하여 이황과 다황 가교 결합을 hexane-1-thiol 과 piperidine 을 chemical probe 로 사용하여 끊고, propane-2-thiol 과 piperidine 을 chemical probe 로 사용하여 다황 가교 결합을 끊어내는 실 험방법을 고안하였다.88 하지만 piperidine 은 유전독성이나 생 식독성 등의 인체에 유해한 영향을 주기 때문에 대체할 수 있 는 아민계열 물질이 필요하였다. Choi 등은 n-hexylamine 을 piperidine 을 대체할 수 있는 아민계열로 이용하여 2% 미만의 낮은 오차율을 갖는 실험결과를 도출하였다.89
단황, 이황, 다황 가교의 가교밀도를 구하는 과정을 Figure 6에 나타내었다. 고무 내 유기물을 THF, n-hexane 을 이용하 여 추출/제거한 후 고무를 건조시킨다. 이후 모든 황 가교에 의한 가교밀도를 toluene 을 이용한 팽윤법을 이용하여 구한다. 단황과 이황 가교에 의한 가교밀도를 구하기 위해서 고무를 미리 팽윤시키고, chemical probe로 propane-2-thiol 과 n-hexylamine을 사용한 toluene 용액에 담가 다황 가교 결합을 끊는다. 다황가교 결합이 끊어진후에는시료를 팽윤 용매인 톨루엔에 담가 잔류 chemical probe 를 제거하고 팽윤시킨다. 톨루엔을 한번 교체하는 것이 바람직하다. 이후 팽윤된 고무 의 무게를 측정한다. 다중 황가교를 끊기 위하여 chemical probe로 propane-2-thiol 대신 hexanethiol 을 이용하고 이후 과 정은 동일하다. 팽윤 전후의 무게를 비교하여 Flory-Rehner 식 을 이용하여 가교밀도를 구한다.
다황 가교를 끊은 가교밀도는 이황과 단황의 가교밀도, 이 황과 다황 가교를 끊은 가교밀도는 단황의 가교밀도이다. 이 를 기반으로 각 황 가교 길이에 의한 가교밀도의 비율는 다 음과 같이 결정한다.
여기서 TS 는 총가교밀도, MS 는 단황의 가교밀도, DS 는 이황 의 가교밀도, PS 는 다황의 가교밀도를 의미한다.
Measurement of Physical Crosslink Density
공유결합에 의한 화학적 가교 이외의 결합을 물리적 가교 결합이라 한다. 고분자는 충진제와의 상호작용에 의해 결합 고무를 생성하며 물리적 상호작용을 한다. Fei 는 같은 가교시 스템에서 카본블랙을가했을때, 카본블랙의 표면과 고분자 간의 접착에 의해 결합고무가 생성되어 가교밀도가 상대적으 로 높음을 보고하였다.89 고분자 내 결정에 의해서도 PE 이나 EVA 와 같이 결정을 생성하는 고분자는 좋은 용매 내에서도 결정이 충분히 풀어지지 않아 상대적으로 가교밀도가 높게 측 정된다.90
Flory-Rehner 식은 충진제가 포함되지 않은 고무에 대한 가 교밀도 식이기 때문에, 충진제를 고려한 가교밀도는 Kraus 식 을 이용하여 나타낼 수 있다.91,92
여기서 Vr0은 충진제가 가해지지 않은 고무의 팽윤된 부피분 율, Vr은 충진제가 가해진 고무의 팽윤된 부피분율, F는 충진 제의 부피분율이다. 충진제가 가해지지 않은 고무의 부피분 율은 오로지 고무의 화학결합을 통한 가교밀도를 나타내고, 충진제가 가해지면 화학결합과 더불어 충진제와 고무사슬의 상호작용이 포함한 가교밀도를 나타낸다. Vr0와 Vr값을 Flory-Rehner 식에 대입하여 총 가교밀도에서 화학적 가교밀도의 차 이로부터 충진제에 의한 고무의 물리적 가교밀도를 구할 수 있다. Hwang은 실리카와 카본블랙을 SBR 에 가하여 Kraus plot을 하였을 때 총 가교밀도와 화학적 가교밀도를 파악하여 충진제의 보강역할을 하는 물질들의 영향을 연구하였다.92 충 진제가 다르게 첨가된 고무의 팽윤된 부피분율은 충진제의 양 에 따라 달라진다. Vr0/Vr을 y축으로, F/(1−F)를 x축으로 하여 도식하였을 때 기울기를 구하여 충진제와 고무사이의 상호작 용 정도를 파악하였다.