Introduction
Research on polymerization using metallocene catalysts has begun since the discovery of ferrocene by Wilkins and Fischer in 1952, and was further developed by Kaminsky in the 1980s. Synthesis of polymers with a narrow molecular weight distribution, high stereo-regularity, and uniform comonomer incorporation is much easier when copolymerization is performed using metallocene catalysts than when using the conventional Ziegler-Natta catalyst. For this reason, many coordination polymerization studies have been conducted using metallocene catalysts.1
Many studies on copolymerization have been pursued for the goal of improving the physical properties of the homopolymers. Chung et al. reported the copolymerization of poly(ethylene-co-p-methylstyrene) using a metallocene catalyst.2,3 Park et al. synthesized a copolymer of ethylene and propylene using a constrained geometry catalyst.4 Quijada et al. prepared a copolymer of ethylene and 1-octadecene using a bridged metallocene, and studied the effect of the comonomer on the catalytic activity.5
As mentioned above, many researchers have studied the catalytic activity, structure, composition, and properties of copolymers under various polymerization conditions. However, most of the studies have been limited to the copolymerization of ethylene and comonomers such as propylene, high α-olefin, diene monomer, cycloolefin, and styrene. There are very few studies on terpolymerization, which can result in various functionalities.6-10
In this study, we synthesized the functional terpolymer, poly(ethylene-ter-high α-olefin-ter-divinylbenzene), which contains a reactive vinyl group. rac-Et(Ind)2ZrCl2 and tri-iso-butylaluminum (TIBA)/dimethylanilinium tetrakis (pentafluorophenyl) borate (DATB) were used as the metallocene catalyst and cocatalyst system, respectively.
Experimental
We used pure nitrogen gas (Dae-myung Gas Co.) after passing it through a molecular sieve (4 Å)/manganese (II) oxide column during all the process. 1-hexene (Aldrich, 97%), 1-octene (Aldrich, 94%), 1-decene (Aldrich, 94%), 1-dodecene (Aldrich, 95%), n-hexane (Samchun pure chemical co., 99.5%), and toluene (Samchun pure chemical co., 99.5%) were distilled through the filter. Inhibitor of divinylbenzene (Aldrich 80%, DVB) was eliminated by washing it with NaOH solution. And then, DVB was distilled under reduced pressure in the presence of CaH2 after performing a standard purification procedure. A solution of rac-Et(Ind)2ZrCl2 (Sigma Aldrich) and dimethylanilinium tetrakis (pentafluorophenyl) borate (Acros) in toluene was also prepared in a glove box. Tri-iso-butylaluminium (Aldrich) was handled under nitrogen atmosphere using a manifolder equipment.
All manipulations were carried out in an inert nitrogen atmosphere. A glove box was used to prevent the contamination of catalysts. All of terpolymerization reaction were carried out in a 300 mL stainless steel autoclave with a mechanical stirrer and a temperature of 50°C. The terpolymerization reaction was initiated by the injection of toluene, high α-olefin, DVB, and solution of catalysts. After 20 minutes, the polymer solution was poured into a dilute HCl/MeOH solution. The resultant polymer was washed with MeOH and dried in vacuo. Each soluble copolymer or terpolymer was separated from the insoluble polyethylene (some types of by-product) using a soxhlet apparatus with n-hexane solvent.
1H-nuclear magnetic resonance (1H-NMR, Bruker AVANCE) analysis was carried out in 60°C at a frequency of 400 and 500 MHz. The sample solutions of the terpolymers were prepared in CDCl3. The deuterated solvent was used to provide an internal lock signal. We calculated the compositions of the terpolymers using 1H-NMR spectra.
The number-averaged molecular weight, weight-averaged molecular weight, and molecular weight distribution of the terpolymers were measured by high-temperature gel permeation chromatography (GPC, Polymer Laboratories Co. PL-GPC210) fitted with Styragel (olesis guard column) HT-type columns. The analyses were performed at 140°C and 1.0 mL/min with 1,2,4-trichlorobenzene as the solvent.
The catalytic activity of the terpolymers was calculated as following equation:
Differential scanning calorimetry (DSC) data for the terpolymer were recorded by means of a DSC7 (PERKIN ELMER Co.). Samples were heated from 0°C to 150°C and then cooled down at 10°C/min to 0°C. Following this, they were reheated at 10°C/min to 150°C. The crystallization temperature (Tc) and melting temperature (Tm) were derived from the second and third run curves, respectively.
Wide-angle X-ray Scattering (WAXS) patterns were recorded in reflection mode at room temperature using D/MAX-2200V X-ray Diffractometer (Rigaku, Tokyo, Japan) connected to a computer. The instrument used the Cu source and performed measurements at 40 kV and 40 mA. The diffraction scans were collected over a period of 20 min between 2θ values from 3.0 to 40.0° at a scan rate of 2°/min.
Results and Discussion
Figure 1 shows the synthetic scheme for the preparation of poly(ethylene-ter-1-hexene-ter-divinylbenzene) (PEHV), poly-(ethylene-ter-1-octene-ter-divinylbenzene) (PEOV), poly(ethylene-ter-1-decene-ter-divinylbenzene) (PEDV), and poly(ethylene-ter-1-dodecene-ter-divinylbenzene) (PEDDV) in the presence of a metallocene catalyst and cocatalyst system. The C2 symmetric rac-Et(Ind)2ZrCl2, was used as the metallocene catalyst. TIBA and DATB were used as cocatalysts. In this system, the active site of the metallocene catalyst is formed via alkylation by TIBA and is activated by the metal ion complex. Polymer chain growth occurs when the metal cationic complex forms a coordinate valence with monomer. The cation of the core metal of the metallocene is also stabilized by interaction with the non-coordinating anion of the bulky cocatalyst containing the borate group.11
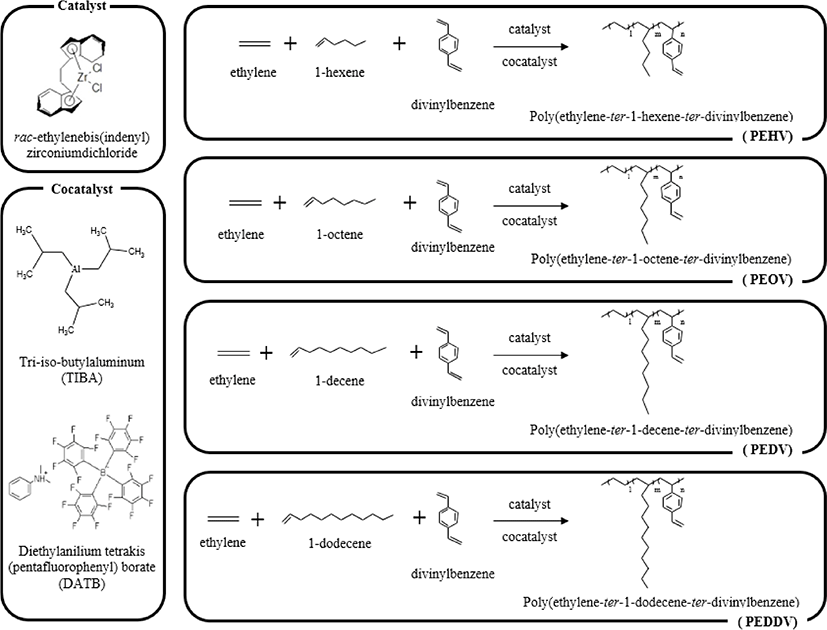
Table 1 summarizes the results of the terpolymerization with different input content of high α-olefins from 0.2 to 0.8 mol/L, while the input content of ethylene and DVB fixed at 0.4 mol/L and 0.2 mol/L, respectively.
Figure 2 shows the catalytic activities of the terpolymers as a function of the input content of high α-olefins. The catalytic activity in the terpolymerization tended to increase with increasing input content of the high α-olefins. This result can be explained by the “comonomer effect” that is well known in coordination copolymerization research.5,12 The high α-olefins incorporated into the main chain reduce its crystallinity, and thus allow for easy diffusion of ethylene through the polymer matrix to the active sites. Incorporation of the comonomer can also change the charge density of the cationic metal ion active site. As a result, the active sites with higher electrophilicity bring about stronger ion pairing between the active site and monomers, which in turn can enhance the catalytic activity and molecular weight. Moreover, comonomers can prevent aggregation around the active site and the cocatalyst.9
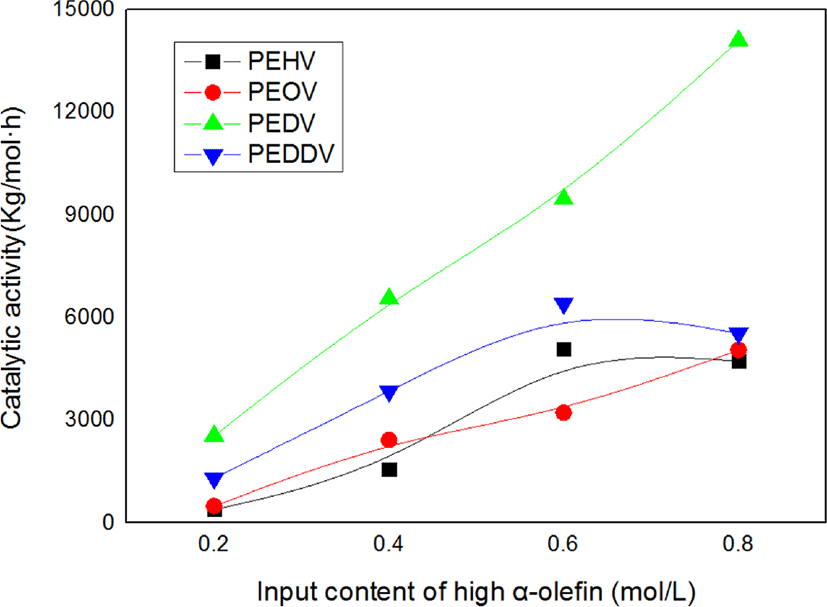
Figure 3 illustrates changes in the weight-average molecular weight of the terpolymers with the input content of high α-olefins. The molecular weight tended to decrease with increasing input content of high α-olefins. It can be reasonably inferred that the number of reaction sites increased due to the improved solvency of the polymerization system owing to the comonomer effect, thereby resulting in a decrease in the molecular weight of the terpolymers.
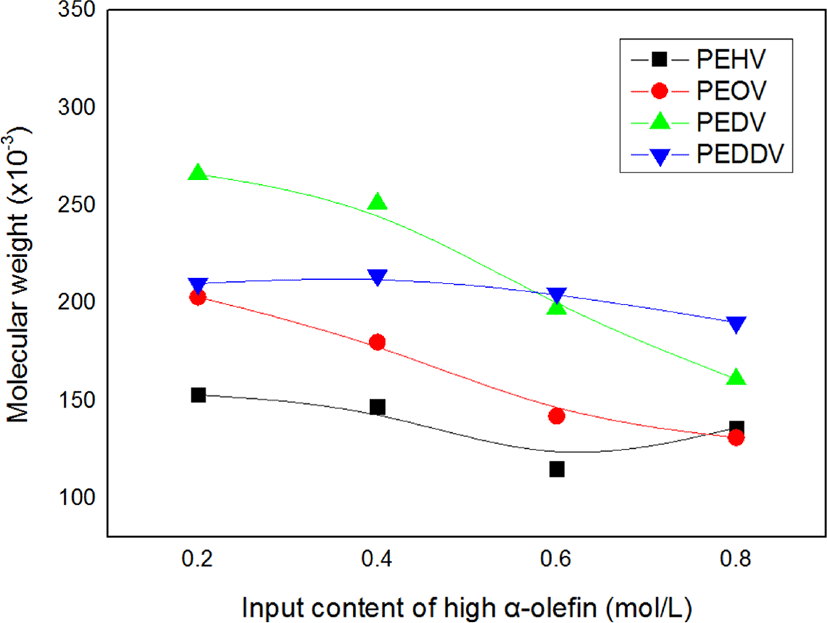
Figure 4 shows the catalytic activity and weight-average molecular weight of various terpolymers having different lengths of incorporated high α-olefins. As the side chain length of the incorporated high α-olefin increased, the catalytic activity and molecular weight increased due to the comonomer effect, which has been mentioned. However, there existed a critical point at which the catalytic activity in the terpolymerization dramatically decreased, i.e., in the case of PEDDV. We assumed that this phenomenon is due to the steric hindrance caused by the very long side chain and increased viscosity around the active metal center.13 These results seem similar to the tendencies observed with our previous study on poly(ethylene-ter-high α-olefin-ter-p-methylstyrene).14,15
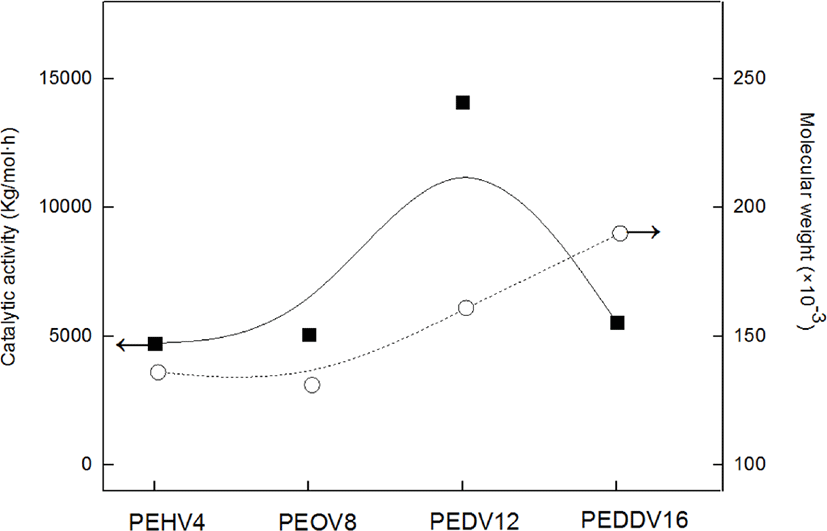
Figure 5 shows the 1H-NMR spectra of the terpolymers, which have different side chain lengths of high α-olefins. The CH2 peaks of ethylene and high α-olefins appeared at 1.00-1.30 ppm. The peaks at 1.00 and 1.50 ppm were attributed to the CH3 group of ethylene and high α-olefins, respectively. Multiple CH peaks attributed to the aromatic ring in DVB appeared at around 7.08 and 7.22 ppm. The vinyl peaks of DVB at around 5.8 ppm confirmed that DVB was successfully incorporated into the terpolymers.
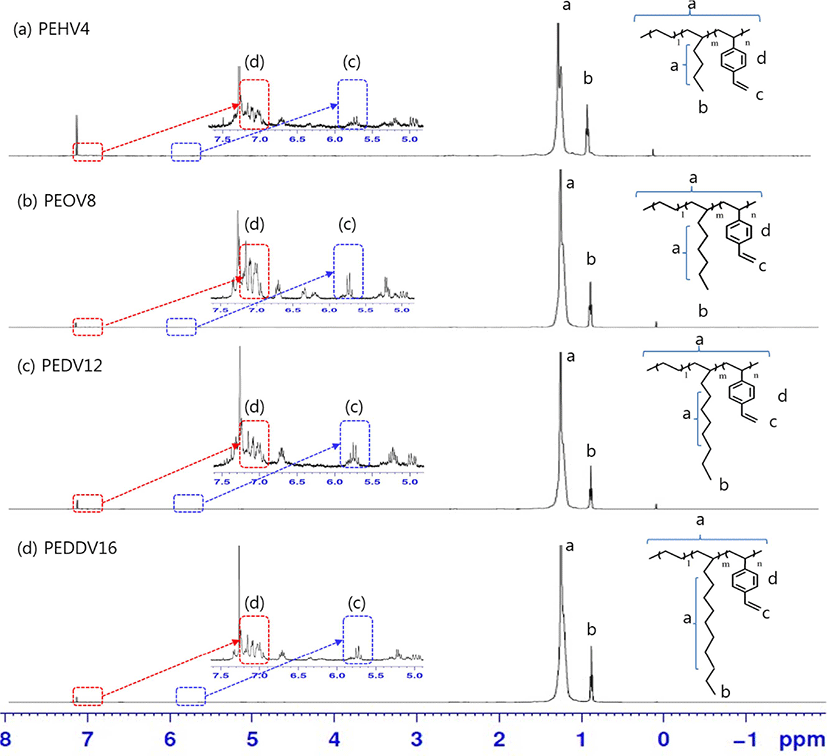
Table 2 shows the composition of the terpolymers depending on the type of high α-olefins and their input amounts. The high α-olefin compositions of PEHV and PEOV gradually increased as the input amounts of the corresponding high α-olefins increased. The DVB compositions of PEHV and PEOV were less than 1.2%, and did not show any clear trend. The monomer composition in the case of PEDV showed no clear trends with the input amount of the high α-olefin. On the other hand, the high α-olefin composition of PEDDV decreased despite the increase in 1-dodecene content, while the composition of ethylene increased, and that of DVB showed no notable trend. We assume that 1-dodecene makes insertion of ethylene easier by reducing the crystallinity around the active sites of the catalysts.
Figure 6 shows WAXS data for the terpolymers. Amorphous peaks were observed around 19.5-20.0°, and crystallization peaks appeared at 21.8° and 24.3°. As the side chain length of the incorporated high α-olefin increased, the crystallinity of the terpolymer decreased. We reasonably infer that the longer chains of the high α-olefin obstruct recrystallization of the terpolymers due to steric hindrance, resulting in a reduction in crystallinity. From the WAXS data in Figure (d), we found that PEDDV is mostly amorphous polymer.
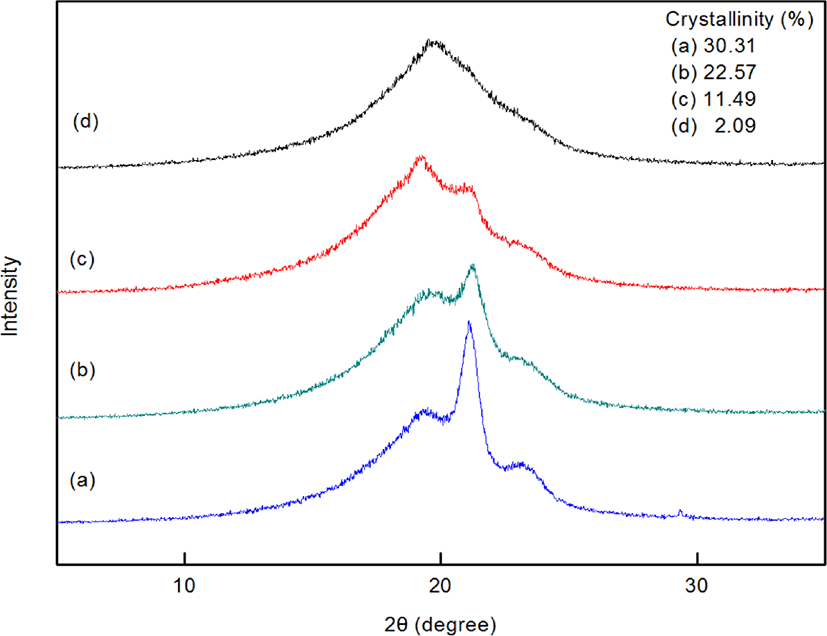
Figure 7 shows the DSC thermograms of the terpolymers. Tm and Tc of the terpolymers were independent of the side chain length of the incorporated high α-olefins. The peaks for Tm and Tc are not clear in the thermograms of PEDDV, because of the lower crystallinity of PEDDV compared with the other terpolymers. This result for PEDDV is in full accordance with the WAXS data.
Conclusions
We synthesized poly(ethylene-ter-high α-olefin-ter-divinylbenzene) using a C2-symmetric rac-Et(Ind)2ZrCl2 metallocene catalyst and cocatalyst system. We prepared the terpolymers by incorporating various high α-olefins such as 1-hexene, 1-octene, 1-decene, and 1-dodecene. The catalytic activity increased with increasing comonomer content. However, the molecular weight showed a tendency to decrease with increasing input content of the high α-olefins. This was due to the increased number of reaction sites resulting from the improved solvency of the terpolymerization system. We also observed that the catalytic activity increased with increasing side chain length of the incorporated high α-olefin. The longer-chain high α-olefin facilitated the diffusion of ethylene through the polymer matrix to the active sites, and changed the charge density of the cationic metal ion active site. However, in the case of PEDDV, the catalytic activity decreased compared with that of PEDV. We assumed that this was caused by the increase in viscosity of the terpolymerization system as well as the steric hindrance due to the very long side chain of 1-dodecene. WAXS data and DSC thermograms indicated that PEDDV has an amorphous structure. In the future, we hope to prepare novel functional graft copolymers by making use of the reactive vinyl group of the terpolymers.