Introduction
천연 고무의 발견과 함께 성장한 고무 산업은 현재 천연 고무를 대체할 다양한 합성 고무들이 개발되어 사용되고 있다. 이렇게 다양한 고무를 바탕으로 보강제, 그리고 sulfur를 비롯한 촉진제의 종류와 함량을 변화시켜 원하는 물성의 고무 제품을 제조하고 있다. Goodyear에 의해 황에 의한 vulcanization 이 발견되었을 때 고무에 대하여 8 wt%의 황을 사용하였고 이 때의 가교 시간은 140°C에서 5시간이 소요되었다. 이후 Oenslager에 의해 aniline에 의한 황의 경화 촉진 효과가 발견되어 가교 시간이 단축되었으며 지속적인 촉진제 개발의 결과 guanidine 촉진제의 개발까지 이어졌다.1 또한 고무의 가공과 가교 조건에 따라 고무 제품의 성능과 물성이 변화되는 것을 알게 되었으며 이에 따른 가공, 가교 조건에 대한 연구가 많아지고 있다. 최근 강화되고 있는 생산성 요구, 제조 기법의 다양화에 맞는 기술 개발을 위하여 고무의 가교 시간 단축에 대한 연구가 활발히 진행되고 있으며 가교 시간 단축의 가장 고전적인 방법으로 가교 온도의 상승을 통한 가교 시간 단축 방법이 사용되고 있다.2 일반적으로 높은 가교 온도는 가교 시간을 단축시키는 효과가 있다고 알려져 있지만 가교 온도 상승에 따른 인장 물성의 저하 및 가교 공정에서의 부적합 발생, scorch time의 감소 등의 부작용이 있기 때문에 적정한 가교 온도의 선정이 매우 중요하다.3,4
최근 지속적인 자동차 산업의 발전에 따라 연비 성능 향상에 대한 요구가 증가하고 있으며, 이에 따른 passenger car radial tire (PCR)의 coefficient of rolling resistance (RRc) 저감에 대한 요구가 지속적으로 증가하고 있다. RRc 저감을 위하여 다양한 polymer의 개발과 carbon black 보강제를 대체하여 silica와 silane의 개발이 진행되었다. 최근 truck bus radial tire (TBR)에서도 coefficient of rolling resistance (RRc) 저감에 대한 요구가 증가하고 있다.5,6 하지만 TB의 경우 마모 성능과 기계적 물성(특히, Cut and Chip 특성)이 매우 중요하기 때문에 green strength가 높은 천연 고무(NR)를 main polymer로 사용하고 있다.7 또한, 기계적 물성의 보강 및 마모 성능이 뛰어난 carbon black을 보강제로 주로 사용하고 있다. 하지만 carbon black을 보강제로 사용할 경우 hysteresis loss에 의한 발열이 많아지는 단점이 있으며, 이러한 단점을 보완하기 위하여 보강제의 일부를 silica로 대체하는 연구가 이루어지고 있다.8 Silica를 일부 대체한 경우 carbon black을 단독으로 사용했을 경우 보다 인열 성능, cut and chip resistance가 향상되는 결과가 보고되어 있다.9,10 하지만 NR의 경우, 큰 분자구조 및 함유하고 있는 단백질/지질들로 인해 silica, silane 그리고 NR간에 화학적 결합을 형성하기 어렵다는 문제점이 있으며, silica를 단독으로 사용할 경우, 내마모성능이 매우 열악해져 TBR의 가혹한 사용 조건에는 적합하지 않다.5 따라서 silica와 carbon black 단독 사용의 단점을 보완하고자 silica와 carbon black을 binary filler system으로 적용하여 내마모성과 점탄성 특성을 동시에 향상시키는 연구가 진행되고 있다.11,12 뿐만 아니라 재료 및 타이어 구조의 변경 이외에 타이어 의 RRc 성능 향상을 위하여 가교 조건의 변경에 대한 연구도 활발히 진행되고 있다.13 특히, 가교 조건은 컴파운드의 물성에 영향을 미치는 중요한 인자이며, 온도에 따른 화학적 vulcanizate structure에 대한 연구가 다양하게 진행되고 있 다.14-17 일반적으로 가교 온도를 낮추게 되면 poly-sulfide vulcanizate structure가 많아지게 되어 점탄성이 향상되는 결과를 나타내었다.14,15 또한 TBR의 경우 천연 고무를 main polymer로 사용하기 때문에 가교 온도를 높이게 되면 scorch 발생 및 reversion 발생에 의한 점탄성 및 기계적 물성이 저하되는 문제가 있어 가교 조건 설정이 매우 어렵고 중요하다.
고무 vulcanizates의 기계적 물성을 결정하는 주요 인자는 고무 내 filler의 분산, filler와 rubber간 interaction, 그리고 sulfur에 의한 가교이다. total crosslink density로 표현되는 vulcanizate structure는 고무 vulcanizates의 기계적 물성에 가장 큰 영향을 미친다. 또한, 컴파운드의 가교 네트워크 정도에 따라 유기 용매 내에서의 equilibrium swelling ratio가 달라진다. 1950년 Flory와 Rehner는 swelling 현상으로부터 unfilled vulcanizate의 total crosslink density를 계산하고 수식화하였다.20,21 Flory-Rehner 식을 Equation (1)에 나타내었다.
ν : crosslink density (mol/g)
Mc : average molecular weight between crosslink points (mol/g)
V1 : volume fraction of rubber in the swollen gel at equilibrium
V0 : molar volume of solvent (cm3/mol)
ρr : density of the rubber sample (g/cm3)
χ : polymer-solvent interaction parameter.
Kraus22와 Boonstra23는 filled vulcanizate에서 carbon black 과 같은 adhering filler가 swelling behavior를 제한시키고 total crosslink density에 영향을 미친다는 것을 확인하였다. 그리고 이는 filler의 volume fraction에 비례하여 나타남을 Equation (2)의 Kraus equation으로 수식화하였다.
vr0 : volume fraction of rubber in the swollen unfilled rubber
vr : volume fraction of rubber in the swollen filled rubber
m : the slope which represents the filler-rubber interaction
φ : the volume fraction of filler.
최근 연구에서는 silica를 filler로 사용한 컴파운드에서의 vulcanizate structure를 정량적으로 silica-silane-rubber에 의한 network와 sulfur에 의한 chemical crosslink 로 구분할 수 있었다.24-28 또한, Figure 1과 같이, carbon black과 silica를 binary filler로 사용하는 컴파운드에서 가교구조를 분류한 연구 결과가 보고되어 있다.29,30 이러한 연구 결과가 발표되기 이전에는 filler-rubber network를 구분할 수 없었기 때문에 가교 온도에 따른 세부적인 vulcanizate structure를 분석하고 가교 온도가 물성에 미치는 영향을 파악하기 어려웠다. 또한 Kim의 이전 연구에서는 PCR에서 주로 사용하는 합성고무인 SBR을 base polymer로 사용하고 carbon black과 silica를 binary filler로 사용한 컴파운드에서 가교 온도에 따른 vulcanizate structure 및 vulcanizate structure와 물성 사이의 상관 관계에 대해 연구하였다.30
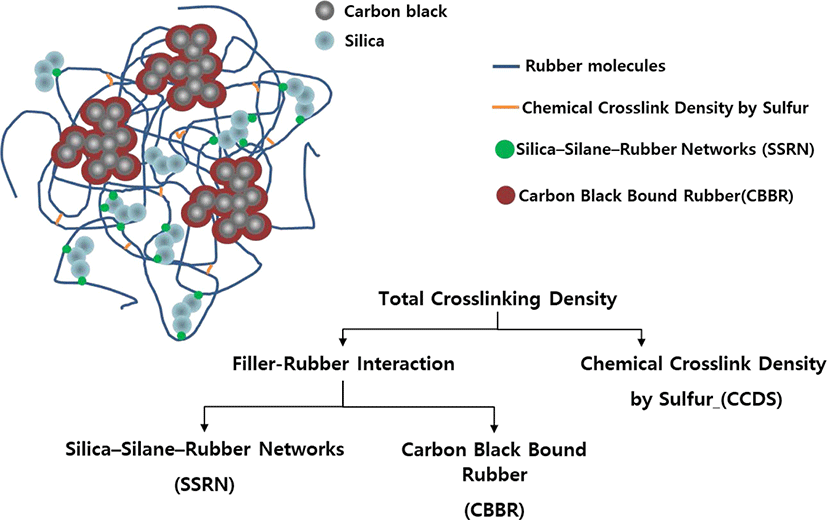
본 연구에서는 TBR에서 주로 사용하는 천연고무(Natural Rubber, NR)을 base polymer로 사용하고 carbon black과 silica를 binary filler로 적용하여 컴파운드를 제조하였다. 가교 온도를 달리하여 vulcanizates를 제조하였으며, vulcanizate structure를 carbon black bound rubber (CBBR)과 silica silane rubber network (SSRN) 그리고 chemical crosslink density of sulfur (CCDS)로 구분하였다. 또한, 타이어 트레드 컴파운드의 성능에 영향을 미치는 물성들을 평가하여 vulcanizate structure와 물성 사이의 상관 관계 및 가교 온도에 따른 민감도를 확인하였다. 본 연구 결과는 NR binary filler system을 사용하는 컴파운드 설계 시 중요한 인자로 작용할 것이다.
Materials and Experimental Methods
본 연구에서는 natural rubber (Cis-1,4-polyisoprene, Standard Indonesian Rubber SIR-20, dirty contents 0.2 wt%)를 base rubber로 사용하였다. Carbon black (N110, OCI Company Ltd., Seoul, South Korea, iodine adsorption: 135-145 g/kg, dibutyl phthalate absorption: 125-135 cm3/100 g)과 silica (Ultrasil 7000 GR, Evonik Industries AG, Essen, Germany, Brunauer–Emmett–Teller (BET) surface area: 160-175 m2/g)를 filler로 사용하였으며, silane coupling agent로 carbon black과 bis-[3-(triethoxysilyl)propyl]tetrasulfide (TESPT)의 1:1 혼합물인 X50-S (Evonik Industries AG, Essen, Germany, silane 50%, carbon black N330 50%)를 사용하였다. 배합재료로 treated distillate aromatic extract (TDAE) oil (Kukdong Oil & Chemicals Co., Ltd., Yangsan, South Korea)을 사용하였으며, 가교 활성화제로 ZnO, stearic acid (all from Sigma-Aldrich Corp.)를 사용하였다. 노화방지제로 N-(1,3-dimethylbutyl)-N’-phenyl-p-phenylenediamine (6PPD, Kumho Petrochemical Co., Ltd., Seoul, South Korea)과 2,2,4-trimethyl-1,2-dihydro-quinoline (TMQ, Sinopec Corp., Beijing, China)를 사용하였다. 가교제로는 sulfur (Daejung Chemicals & Metals Co., Ltd., Siheung, South Korea)를 사용하였으며 촉진제로 N-tert-butyl-2-benzothiazyl sulfonamide (TBBS, Shandong Yanggu Huatai Chemical Co., Ltd., Liaocheng, China)와 1,3-diphenyl guanidine (DPG, Merck KGaA, Darmstadt, Germany)을 사용하였다. Scorch 방지를 위한 가교 지연제(pre-vulcanization inhibitor)로 N-(cyclohexylthio)phthalimide (Shandong Yanggu Huatai Chemical Co., Ltd., Liaocheng, China)를 사용하였다.
Vulcanizate structure 분석을 위하여 filler를 종류별로 변량하여 컴파운드를 제조하였으며 배합 formulation을 Table 1에 나타내었다. Table 1에 나타낸 formulation에 따라 kneader (MSI-IRM 300, 300 cc, Mirae SI Co. Ltd., Gwangju, Korea)를 이용하여 배합을 실시하였다. Silane coupling agent인 TESPT는 silica 함량에 8 wt%를 계산하였다. 실제 사용된 X50-S는 50%의 TESPT를 함유하고 있어 실제 16 wt%를 사용하였다. 또한 X50-S는 입자 크기가 큰 N330을 50% 포함하고 있으나, 본 연구에서 사용한 carbon black은 입자 크기가 작은 N110을 사용하였기에 실제 carbon black과 silica의 비율 계산에서는 제외하였다. 컴파운드는 kneader에서 70%의 fill factor로 10분 40초 동안 배합하였다. 1st stage와 2nd stage에서 mixing 초기 온도는 각각 110°C, 50°C로 설정하였고 mixing 완료 후 dump 온도는 각각 150-155°C 와 100°C 로 제어하였다. 1st stage에서 silica와 silane coupling agent는 각각 50%씩 분할하여 투입하였다. 이는 mixer의 용량이 작아 silica와 silane coupling agent의 손실을 최소화하기 위함이다. 상세한 mixing 순서는 Table 2에 나타내었다.
배합물은 moving die rheometer (MDR)를 이용하여 ± 1°의 vibration angle 로 150°C, 160°C, 170°C에서 미가황 고무 시편의 torque값과 최적의 가교 시간(t90)을 측정하였다. 이후 150°C, 160°C, 그리고 170°C의 유압 고온 프레스에서 t90 시간 동안 가압하여 vulcanizates를 제조하였다. 제조된 vulcanizates은 universal testing machine (UTM, KSU-05M-C, KSU Co., Ansan, Korea)을 이용하여 컴파운드의 인장 물성(Modulus, 인장 강도, 연신율)을 측정하였다. 인장 물성 결과는 각각 3번 측정한 후 평균값을 사용하였다. 인장 시험은 ASTM D412 시험법을 사용하였으며 500 N의 load cell로 500 mm/min의 속도를 적용하였다. 동적 점탄성을 측정하기 위하여 ARES-G2 (TA instruments, Delaware, USA)를 사용하였으며 시험 조건은 0.5% strain, 10 Hz frequency, torsion mode 그리고 온도는 −60°C 부터 60°C 로 설정하여 측정하였다.
가교된 시편을 10 mm × 10 mm × 2 mm size로 준비하였다. 시편 내의 유기물을 제거하기 위하여 30 ml THF와 n-hexane을 사용하여 각각 2일 동안 상온에서 침지시켰다. 유기물이 제거된 시편을 40°C vacuum oven에서 3일간 건조시켰다. 건조 시편을 toluene에 침지시켜 25°C에서 24시간 동안 보관하여 팽윤시켰다. total crosslink density를 계산하기 위하여 팽윤 전, 후의 무게를 측정하여 팽윤된 고무의 부피 분율을 계산하였다. Swelling Test (3)와 Flory-Rehner equation (1)과 Kraus equation (2)을 이용하여 vulcanizate structure를 분석하였다.
wdry : the weight of dry sample
wfiller : the weight of filler in dry sample
wswollen : the weight of swollen sample
ρrubber : the density of rubber
ρsolvent : the density of solvent.
Boonstra 와 Kraus22,23의 연구에 따르면 vr0/vr 의 비율 즉 unfilled 상태의 팽윤된 고무의 부피 분율과 filled 상태의 팽윤된 고무의 부피 분율의 비율이 filler의 함량을 증가시키면 선형적으로 감소한다고 알려져 있다. 이는 filler-rubber interaction의 차이에 의해 팽윤비가 달라지기 때문이다. 따라서 Kraus 식 (2)에서 m 값이 filler-rubber interaction을 나타낸다고 할 수 있다. 또한 Flory-Rehner식에서 vr 값으로 total crosslink density를 확인 할 수 있으며 vr0을 통하여 unfilled vulcanizates의 crosslink density를 구할 수 있다. 따라서 total crosslink density에서 filler-rubber interaction과 chemical crosslink density를 정량적으로 구분할 수 있다. Lee의 연구에서 swelling test와 Equations (1) 과 (2)를 이용하여 silica 또는 carbon black이 filled vulcanizates에서의 total crosslink density와 filler-rubber interaction 그리고 chemical crosslink density를 구분하였다.24 게다가 Kim의 이전 연구에서는 carbon black과 silica가 binary filler로 충진된 ESBR 컴파운드에서 swelling test와 Equations (1)과 (2)를 이용하여 carbon black bound rubber (CBBR)과 silica silane rubber network (SSRN) 그리고 chemical crosslink density of sulfur (CCDS)를 구분하였으며 이를 Figure 2에 나타내었다.29,30 본 연구에서는 Kim의 이전 연구와 동일한 방법으로 vulcanizate structure를 분석하였으며 가교 온도를 변경에 따른 vulcanizate structure 분석을 추가로 실시하였다.
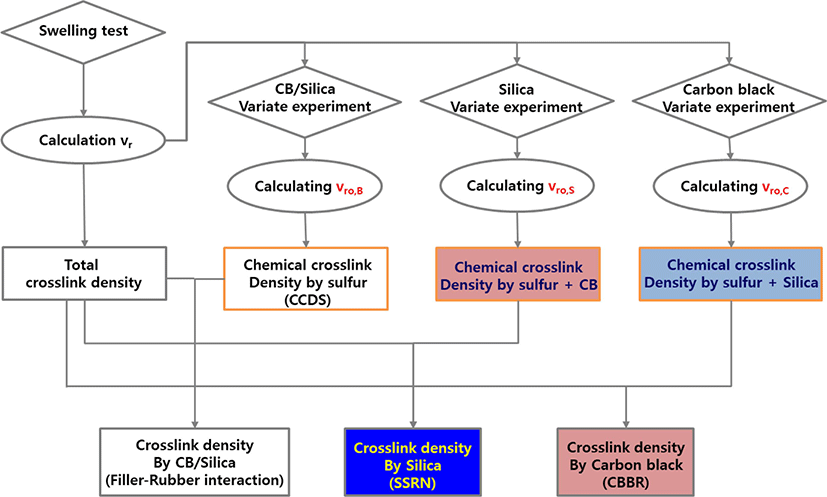
Results and Discussion
Carbon black 60phr, silica 30phr이 투입된 T-3 compound의 가교 온도를 각각 150°C, 160°C, 170°C 로 변화시켜 얻은 cure curves와 가황 특성을 Figure 3과 Table 3에 나타내었다. 가교 온도가 150°C에서 170°C 로 상승함에 따라 가교 시간 (t90)이 4분가량 짧아지고, 이를 통해 가교 시간 단축에 따른 생산성 향상을 기대할 수 있다. 하지만, 압출 및 압연 등의 가공 공정에서의 scorch 발생을 예측할 수 있고, 가교 공정에서의 고무의 flow를 예측할 수 있는 scorch time (t10)이 약 1분 감소하는 것을 확인할 수 있다. 천연 고무를 base polymer로 사용한 컴파운드의 경우 scorch time이 짧은 것이 특징이며 이는 가교 공정에서 컴파운드의 flow성 저하 및 scorch로 인하여 고무가 mold에 완전히 채워지지 않아 발생하는 light성 부적합을 발생시킬 수 있다. 가교 온도에 따른 total crosslink density를 예측하는 maximum torque (Tmax)값과 ΔT (Tmax – Tmin)을 확인한 결과, Tmax와 ΔT 모두 감소하는 경향을 나타내었으며, 이는 가교 온도 상승에 따른 total crosslink density의 감소를 예측할 수 있다. 또한 170°C 가교 조건에서는 Tmax 도달 이후 torque 값이 저하되는 reversion 현상이 더욱 뚜렷하게 나타난다. 따라서 천연 고무를 main polymer로 사용한 컴파운드에서는 가교 온도 상승에 따른 가교 시간 관리가 매우 중요하다. Filler 함량별 가교 온도에 따른 ΔT를 Figure 4에 나타내었다. Filler의 종류에 관계없이 가교 온도 상승에 따라 ΔT가 감소하였고, 이는 CBBR, SSRN, CCDS 이 모두 감소하여 total crosslink density 가 감소하였기 때문으로 판단된다. Vulcanizate structure별 crosslink density에 대해서는 section 3.2에서 더 자세히 다루도록 한다. 또한 silica 함량 및 carbon black 함량 증가에 따라 ΔT 값은 증가하였으며 이는 total crosslink density의 증가에 기인한 것으로 판단된다. 150°C 가교 조건에서는 marching 현상이 발생함에 따라 ΔT가 상승하였다. 160°C 가교 조건에서는 plateau cure curves를 나타내지만, 170°C 가교 조건에서는 polysulfide group 이 파괴되면서 Tmax 값이 낮아지는 reversion이 발생하였다. 이러한 현상은 평가된 모든 컴파운드(T-1 ~ T-7) 가 동일한 경향을 나타내었으며, 본 연구에서는 최적 crosslink density를 얻고 reversion에 의한 물성 변화를 방지하기 위하여 최적 가교 시간(t90) 동안 시편을 가교하여 인장 물성, 점탄성에 대한 분석을 실시하 였다.
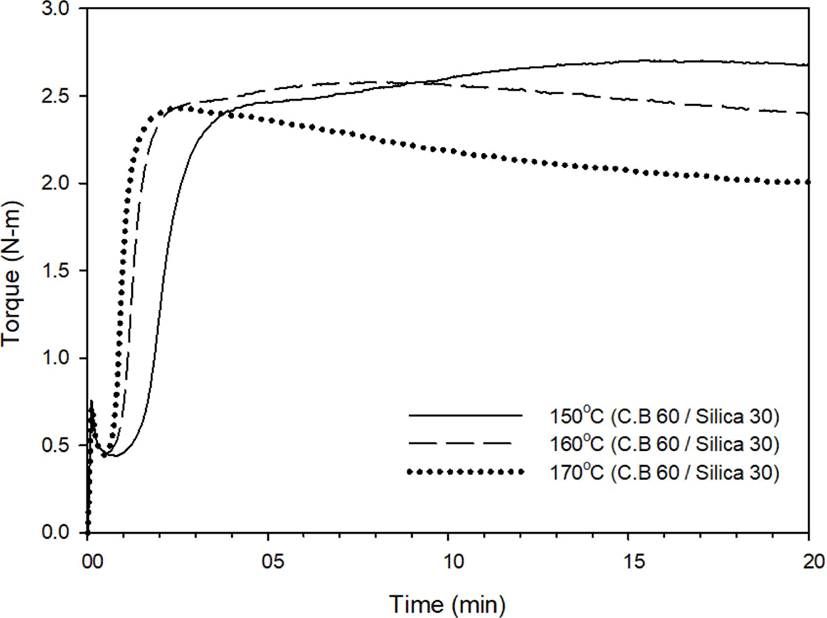
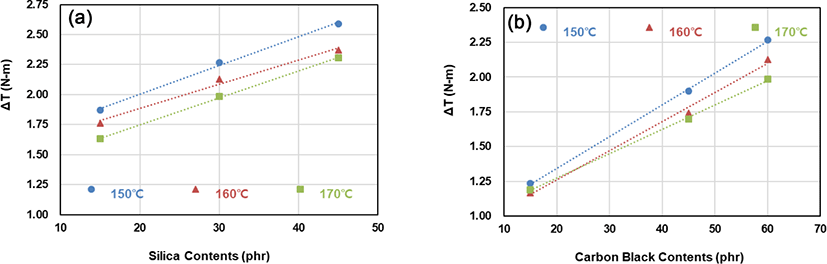
Vulcanizate structure를 분석하기 위하여 filler 함량이 다른 컴파운드를 이용하면 total crosslink density 내에서 변량한 filler에 의한 filler-rubber interaction과 변량 하지 않은 filler에 의한 filler-rubber interaction 및 sulfur에 의한 chemical cross-link density의 합으로 구분할 수 있다. 본 연구에서는 가교 온도에 따른 vulcanizate structure를 분석하기 위해 filler 함량을 변량한 T-1에서 T-7 컴파운드를 150°C, 160°C, 170°C의 가교 온도에서 가교 한 후 vulcanizate structure를 분석하였다. vulcanizate structure 분석 결과를 Table 4와 Figure 5에 나타내었다. Figure 2에 제시된 scheme에 따라 silica 함량을 30phr로 고정하고 carbon black 함량을 변량한 T-1, T-2, T-3 컴파운드를 150°C에서 가교 하여 계산된 CBBR 값(6.40 × 10−5 mol/g)과 carbon black 함량을 60phr로 고정하고 silica 함량을 변량한 T-3, T-4, T-5 컴파운드를 150°C에서 가교 하여 계산된 SSRN 값(3.08 × 10−5 mol/g)의 합이 silica와 carbon black을 동시에 변량한 T-3, T-6, T-7에서 계산된 filler-rubber interaction의 값(9.48 × 10−5 mol/g)과 일치하였다. 따라서, ESBR (emulsion styrene butadiene rubber) binary filler system 이 아닌 NR binary filler system에서도 CBBR, SSRN, CCDS 로 vulcanizate structure를 구분할 수 있음을 증명할 수 있었다. 160°C, 170°C에서도 동일한 방법으로 vulcanizate structure를 구분하였고, T-3 컴파운드의 vulcanizate structure별 crosslink density와 그 변화율을 Table 4와 Figure 6에 나타내었다.
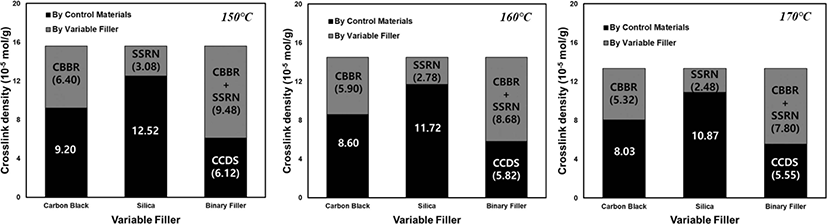
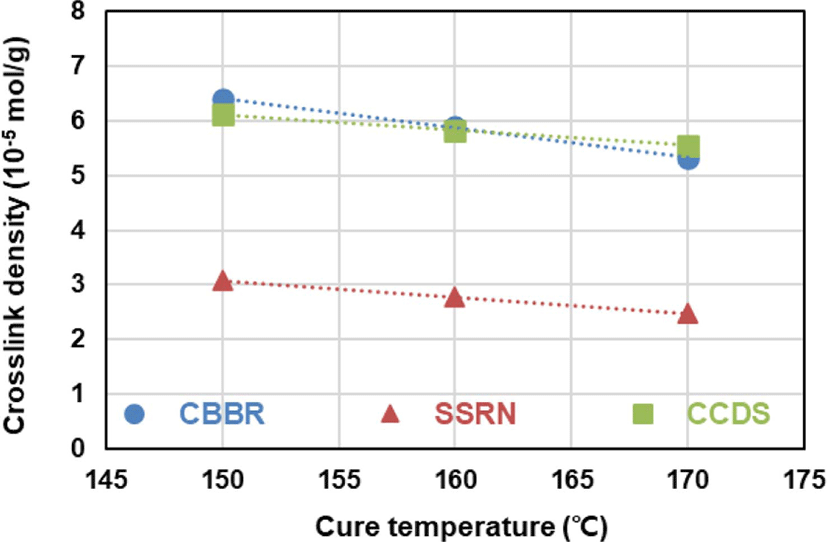
첫째, 가교 온도가 상승함에 따라 CBBR, SSRN, CCDS 모두 감소하는 경향을 나타내었으며 total crosslink density 또한 감소하는 것을 확인하였다. 특히, NR binary filler system 에서의 decrease rate (−0.113 × 10−5 mol/g°C) 가 ESBR binary filler system (−0.101 × 10−5 mol/g°C) 대비 조금 더 큰 값을 나타내었다.30 이는 ESBR 대비 NR의 낮은 열안정성으로 인해 crosslink density 가 감소하였기 때문이다.
둘째, ESBR binary filler system에서는 CBBR 이 −0.05 × 10−5 mol/g°C 의 decrease rate 를 나타내어 −0.027 × 10−5 mol/g°C 과 −0.024 × 10−5 mol/g°C 의 decrease rate 를 나타낸 SSRN, CCDS 대비 약 2배의 decrease rate 차이를 보였다.30 NR binary filler system 역시 CBBR 이 −0.054 × 10−5 mol/g°C 의 decrease rate을 나타내어 −0.030 × 10−5 mol/g°C 과 −0.029 × 10−5 mol/g°C 의 decrease rate을 나타낸 SSRN 과 CCDS 대비 약 2배의 decrease rate 차이를 보였다. 이는 SSRN과 CCDS의 chemical network가 CBBR의 physical network 보다 결합력이 강하여 가교 온도 증가에 따른 crosslink density의 감소가 적었기 때문이라 판단된다.
셋째, ESBR binary filler system에서는 가교 온도 150°C, 160°C 그리고 170°C에서 SSRN의 값과 비율이 각각 3.35 × 10−5 mol/g°C (20.0%), 3.17 × 10−5 mol/g°C (19.9%), 2.82 × 10−5 mol/g°C (19.1%)의 값을 나타내었으나 NR binary filler system에서는 각각 3.08 × 10−5 mol/g°C (19.7%), 2.78 × 10−5 mol/g°C (19.2%), 2.48 × 10−5 mol/g°C (18.6%)의 값을 나타내었다. 다시 말해, NR binary filler system에서의 SSRN이 ESBR binary filler system 대비 약 0.3 × 10−5 mol/g 낮은 것을 알 수 있다. 이는 NR의 단백질, 지질로 인해 silanization 효율이 낮아짐에 따라 silica-silane-NR 간의 coupling 효과가 낮아졌기 때문이라 판단된다.5 반면, CBBR의 경우, NR binary filler system에서 6.40 × 10−5 mol/g (41.0%), 5.90 × 10−5 mol/g (40.7%), 5.32 × 10−5 mol/g (39.9%) 값을 가져, ESBR binary filler system에서의 6.21 × 10−5 mol/g (37.0%), 5.82 × 10−5 mol/g (36.5%), 5.21 × 10−5 mol/g (35.2%) 와 유사한 값을 나타내었다. 이는 단백질과 지질이 hydrophobic 한 carbon black 과 rubber 사이의 physical network 에는 큰 영향을 주지 못하기 때문이라 판단된다. 또한, NR binary filler system에서는 가교 온도 150°C, 160°C, 170°C에서 CCDS 가 6.12 × 10−5 mol/g, 5.82 × 10−5 mol/g, 5.55 × 10−5 mol/g 값을 가져, ESBR binary filler system에서의 7.22 × 10−5 mol/g, 6.95 × 10−5 mol/g, 6.75 × 10−5 mol/g 대비 약 1.1 × 10−5 mol/g 정도 낮은 값을 보였다. 이는 NR 내에 포함되어 있는 단백질, 지질이 silanization을 방해하여 silica 표면에 촉진제가 쉽게 흡착됨에 따라 CCDS 가 낮아졌다고 판단된다. 또한 가교 온도 상승에 따라 CBBR의 감소율(−0.054 × 10−5 mol/g°C)이 CCDS의 감소율(−0.029 × 10−5 mol/g°C) 대비 크고 total crosslink density 내에서 CCDS가 차지하는 비율이 39.2%에서 41.6%로 2.4% 증가함을 알 수 있다.
Binary filler system 내 vulcanizate structure에서 CBBR과 SSRN을 carbon black과 silica 함량으로 나누어 각각의 filler 1phr이 crosslink density에 기여하는 값을 계산하였고 이를 Table 5에 나타내었다. NR binary filler system 에서는 가교 온도에 상관없이, carbon black 1phr 이 증가시키는 CBBR 은 silica 1phr 이 증가시키는 SSRN 보다 더 컸으며, 이는 ESBR binary filler system 결과와는 반대되는 경향이다.30 이는 NR과 silica 사이의 coupling reaction 효율이 ESBR binary filler system 대비 낮아졌기 때문이라 판단된다. 또한 가교 온도 증가에 따른 CBBR/phr의 감소율 −0.0117 × 10−6 mol/g°C은 SSRN/phr의 감소율 −0.0083 × 10−6 mol/g°C 보다 컸으며, 이는 앞서 설명하였듯이 SSRN의 강한 chemical network 로 인해 가교 온도 증가에 따른 SSRN의 감소가 적었기 때문이라 판단된다.
Description (10−6 mol/g) | 150°C | 160°C | 170°C | Decrease rate (10−6 mol/g∙°C) |
---|---|---|---|---|
CBBR/phr | 1.17 | 1.05 | 0.93 | -0.0117 |
SSRN/phr | 1.03 | 0.97 | 0.87 | -0.0083 |
Section 3.2에서 가교 온도 변화에 따라 filler 1phr이 crosslink density에 기여하는 값을 계산하였다. 가교 온도 변화에 따라 filler 1phr의 crosslink density 기여도 CBBR/phr과 SSRN/phr이 modulus at 100% elongation (M100%)에 미치는 영향을 Table 6와 Figure 7(a)에 나타내었다. 가교 온도에 따른 vulcanizates의 stress-strain curves는 Figure 8에 나타내었다. 특히 저신장 영역에서의 stress 값인 M100%에 미치는 영향은 가교 온도에 관계없이 SSRN/phr이 CBBR/phr보다 큰 것을 알 수 있다. 이는 SSRN의 저신장 영역에서의 강한 filler-rubber interaction에 의한 것으로 판단된다.31 또한 가교 온도가 상승함에 따라 SSRN/phr 과 CBBR/phr이 M100%에 미치는 영향이 증가하였는데, 앞서 section 3.2에서 언급한 가교 온도가 상승함에 따라 CBBR, SSRN, CCDS 모두 감소하는 경향과 반대되는 결과를 나타내었다. 이는 가교 온도가 상승함에 따라 filler-rubber interaction (CBBR+SSRN) 이 차지하는 비율이 150°C 에서는 60.7%였으나 170°C 에서는 58.6%로 2.1% 감소하였으며, CCDS는 150°C에서 39.2%였으나 170°C에서는 41.6%로 약 2.4% 증가하였기 때문이라 판단된다. 가교 온도 상승에 따른 M100%의 변화율(RoC)은 SSRN이 CBBR 대비 크게 나타났다. 이는 silica와 silane의 silanization 이후 NR과의 coupling 반응이 어려운데, 가교 온도를 높여 주어 coupling 반응 증가에 의한 SSRN의 강한 filler-rubber interaction 형성에 기인한 것으로 판단된다.
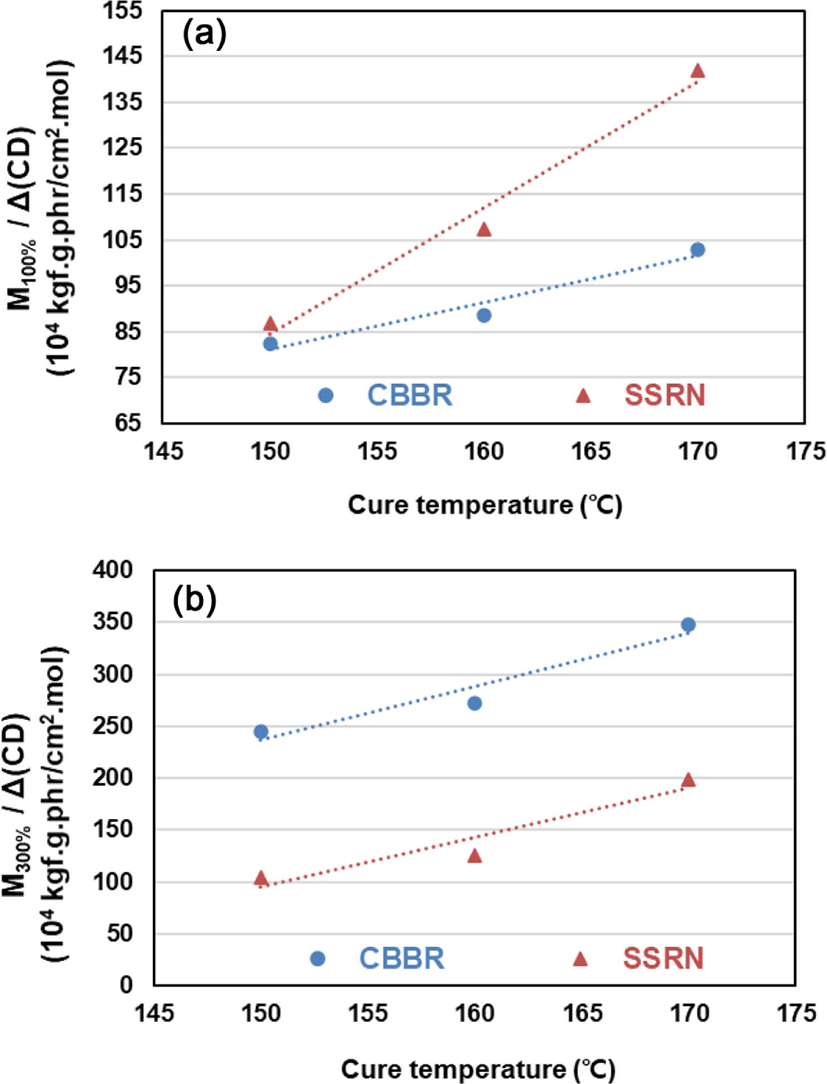
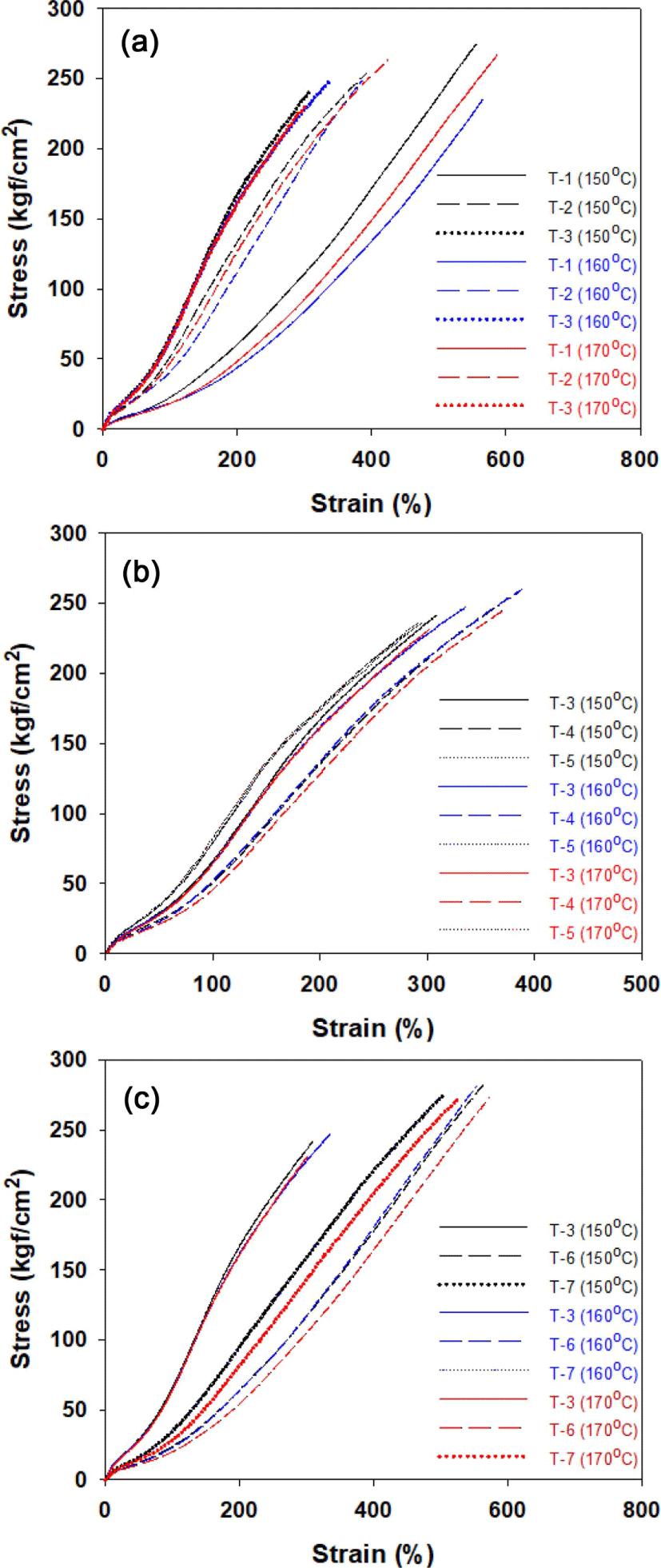
가교 온도에 따라 filler 1phr의 crosslink density 기여도 CBBR/phr과 SSRN/phr이 modulus at 300% elongation (M300%)에 미치는 영향을 Table 6와 Figure 7(b)에 나타내었다. 고신장 영역인 M300%에 미치는 영향도는 가교 온도에 관계없이 CBBR/phr이 SSRN/phr 보다 2배 이상 큰 것을 알 수 있다. 이는 고신장 영역에서 silica-silica network 가 파괴되는 반면31 carbon black의 경우 고신장 영역에서 고무와 강한 physical interaction을 유지하기 때문이다.32 가교 온도가 상승함에 따라 SSRN/phr과 CBBR/phr의 영향도가 증가하는 것을 알 수 있으며 이는 M100% 와 동일한 결과로 가교 온도가 상승함에 따라 filler-rubber interaction (CBBR+SSRN) 이 크게 감소하여 CCDS 가 total crosslink density에 미치는 영향이 증가하였기 때문이다. 그리고 가교 온도 상승에 따른 M300%의 변화율(RoC)은 CBBR이 SSRN 대비 크게 나타났다. 이는 CBBR이 SSRN에 비해 M300%에 미치는 영향도가 크기 때문이라 판단된다.29 고무가 silica 와 커플링 반응을 통해 화학 결합을 하더라도 100% 이상의 strain에서는 화학 결합의 영향이 약해진다. 하지만 carbon black에 흡착한 고무는 physical bond를 형성하고 있고 glassy한 bound rubber에 의하여 더 높은 crosslink density를 나타낸다. 특히 Fukahori의 interface model에 따르면, bound rubber는 glassy state의 내부 polymer layer와 이보다는 덜 단단한 외부 polymer layer의 이중 layer 구조로 구성되어 있다.27 따라서, 고신장 영역에서는 외부 polymer layer의 rubber molecules가 연신 방향으로 분자 strand가 배향되어 높은 보강 효과를 나타낼 수 있다.
타이어 트레드의 성능은 컴파운드의 dynamic viscoelasticity 와 우수한 상관관계를 가진다. 특히, complex modulus (G*) at −20°C, loss modulus (G’’) at 0°C, 그리고 tan δ at 60°C은 각각 snow traction, wet traction, 그리고 rolling resistance의 indicator로 널리 사용되고 있다.33-36 NR vulcanizates with binary filler system에서 가교 온도 상승에 따라 filler 1phr의 crosslink density 기여도 CBBR/phr과 SSRN/phr이 snow traction, wet traction, 그리고 rolling resistance에 미치는 영향을 분석하였으며 이를 Table 7과 Figure 9에 나타내었다. Rolling resistance를 나타내는 tan δ at 60°C 값은 낮을수록 RR에 유리하다고 알려져 있다.37-39 가교 온도와 상관없이 SSRN/phr에 의한 tan δ at 60°C 값의 변화는 적었고, CBBR/phr 대비, RR에 더 긍정적인 영향을 나타내었다. 하지만 가교 온도가 증가할수록 CBBR/phr에 의한 tan δ at 60°C 값은 점차 증가하여 RR에 불리한 영향을 나타내었다. 이는 section 3.2에서 언급하였듯, 가교 온도가 상승함에 따라 CBBR, SSRN, CCDS 모두 감소하여 total crosslink density가 감소하였기 때문이라 판단된다. 특히 가교 온도 상승에 따라 CBBR의 tan δ at 60°C 변화율이 SSRN의 tan δ at 60°C 변화율 대비 큰 것을 알 수 있다. 이는 carbon black과 rubber 사이의 physical network인 CBBR이 chemical network 인 SSRN과 CCDS 대비하여 0.5% strain, 10 Hz의 조건에서 가교 온도에 의한 결합 파괴가 더 크기 때문이다.
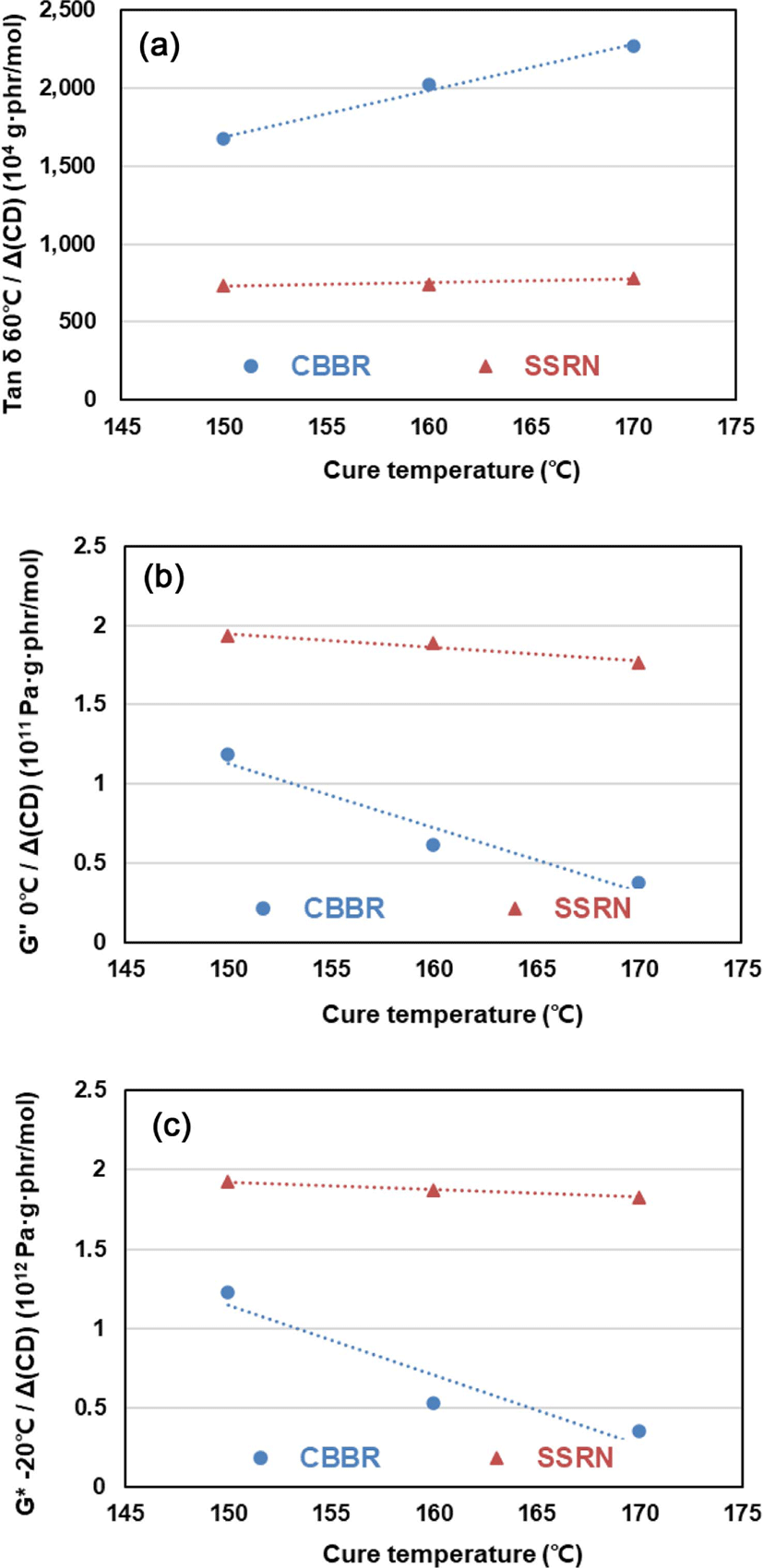
Wet traction을 예측할 수 있는 G’’ at 0°C에 대한 filler 1phr의 crosslink density 기여도와 변화율을 가교 온도에 따라 분석하였다. 가교 온도에 상관없이 SSRN/phr이 CBBR/phr 대비 G” at at 0°C 값에서 높은 값을 나타내었다. 점탄성 특성상 G” at 0°C 값이 높을수록 wet traction에 유리하다고 알려져 있으며 본 연구 결과, filler 1phr 당 crosslink density의 기여도 (contribution of crosslink density per unit content of filler)는 SSRN/phr 이 CBBR/phr 보다 wet traction에 더 큰 영향을 미친다고 판단된다. 가교 온도에 따라 SSRN에 의한 G” at 0°C 영향도는 감소하였으나 그 변화율이 작았다. 하지만 CBBR에 의한 G” at 0°C 영향도는 크게 감소하는 결과를 나타내었다. 이는 가교 온도에 따른 carbon black과 silica에 의한 vulcanizate structure의 변화보다 filler의 특성에 따른 영향이 큰 것으로 판단된다.
G* at −20°C 값은 낮을수록 snow traction에 유리하다고 알려져 있다. 가교 온도에 상관없이 SSRN/phr이 CBBR/phr 대비 높은 G* at −20°C 값을 나타내었으며, filler 1phr당 crosslink density의 기여도는 SSRN/phr 이 CBBR/phr 보다 snow traction에 더 큰 영향을 미친다고 판단된다. 그리고 가교 온도 상승에 따라 SSRN에 의한 G* at −20°C 영향도는 소폭 감소하고 그 변화율이 작았다. 하지만 CBBR에 의한 G* at −20°C 영향도는 크게 감소하는 결과를 나타내었다. NR binary filler system에서는 filler에 의한 wet traction과 snow traction에 미치는 영향은 SSRN이 CBBR 대비 크며, 이는 Kim et al.의 이전 연구 ESBR binary filler system의 연구 결과와 일치하는 것이다.29,30
Conclusions
본 연구에서는 NR binary filler system에서의 가교 온도에 따라 컴파운드의 vulcanizate structure를 CBBR, SSRN 그리고 CCDS로 분류하고 정량화 하였다. 또한 가교 온도에 따라 각각의 vulcanizate structure가 물성에 미치는 영향을 분석하였다. CBBR 은 carbon black과 rubber 사이의 physical network 로 이루어져 있으며, SSRN과 CCDS는 chemical network 로 이루어져 있다. vulcanizate structure 분석 결과, 가교 온도가 증가함에 따라 CBBR, SSRN, CCDS 모두 감소하는 경향을 나타내었으며, 이는 ESBR binary filler system과 동일한 결과이다. 하지만 NR은 ESBR 대비 낮은 열안정성으로 인해 crosslink density의 감소율이 NR binary system에서 크게 나타났다. 또한 NR binary filler system에서의 CBBR의 감소율이 SSRN과 CCDS에 의한 감소율 대비 2배 차이를 나타내었으며 이는 SSRN의 화학적 결합이 CBBR의 physical network인 bound rubber의 결합력보다 강하기 때문이다. 특히 NR binary system에서의 SSRN 값이 ESBR binary filler system 대비 낮은 값을 나타내었는데 이는 NR의 특성에 따른 silanization 과 coupling효율의 저하에 의한 것으로 판단된다. 이에 따라 filler 1phr이 filler-rubber interaction에 미치는 영향도에서도 silica (SSRN/phr) 대비 carbon black (CBBR/phr) 이 큰 값을 나타내었으며 이는 ESBR binary filler system 과는 반대되는 경향이다.29,30 가황 특성 분석 결과 filler의 종류에 상관없이 가교 온도 상승에 따라 ΔT가 감소하는 결과를 나타내었다. 이는 NR binary filler system에서 가교 온도의 상승에 따라 CBBR, SSRN, CCDS 모두 감소하여 total crosslink density 가 감소하였기 때문으로 판단된다. 기계적 물성 평가 결과, 100% modulus는 SSRN의 영향이 크고, 300% modulus는 CBBR의 영향이 큰 것으로 나타났다. 또한 가교 온도가 상승함에 따라 100% modulus, 300% modulus 모두 SSRN/phr 과 CBBR/phr의 영향도가 증가하였다. 이는 가교 온도가 상승함에 따라 filler-rubber interaction (CBBR+SSRN) 이 크게 감소하여 CCDS 가 total crosslink density에 미치는 영향이 증가하였기 때문이다. 점탄성 분석 결과, tan δ at 60°C에 미치는 절대적인 영향은 SSRN이 CBBR 대비 우수하였다. 하지만 가교 온도를 증가함에 따른 filler-rubber interaction의 감소율이 SSRN 대비 CBBR이 크기 때문에 CBBR 이 tan δ at 60°C 값을 더 크게 증가시켰으며 이는 ESBR binary filler system과 동일한 연구 결과이다.30 NR binary filler system에서는 filler에 의한 wet traction과 snow traction에 미치는 영향은 SSRN이 CBBR 대비 크며 이는 silica filler의 고유 특성에 의한 결과로 판단된다. 따라서 본 연구에서 NR binary filler system (carbon black/silica)에서 가교 온도에 따른 vulcanizate structure 분석 결과를 통하여 ESBR binary filler system과 차이점을 확인하였으며, NR binary filler system에서 crosslink density를 효과적으로 증가시키고 요구하는 인장 물성 및 점탄성을 나타내기 위한 guide를 제시하고자 한다. 가교 온도와 carbon black/silica의 비율에 따른 컴파운드의 물성 변화의 경향을 Table 8에 나타내었다. 이렇게 제시된 결과는 실제 컴파운드 formulation설계 시 filler의 사용량 및 종류를 결정하고 가교 조건 설정에 중요한 지침으로 활용될 수 있을 것이다.