Introduction
Poly(ethylene-co-vinyl acetate) (EVA) is a copolymer of ethylene and vinyl acetate (VA), and the VA units are randomly distributed throughout the ethylene polymer backbone. EVA is a transparent thermoplastic copolymer, and it becomes more flexible and transparent by increasing the VA content. EVA has crystalline structures due to the ethylene sequences, and its properties and application fields are mainly depending on the VA content.1-6 The crystalline structures are influenced by the VA units (Scheme 1). Crystallinity and melting points of EVA decrease with increase in the VA content.7 X-ray diffraction (XRD) and differential scanning calorimetry (DSC) are common analysis techniques to investigate crystalline properties of polymeric materials.8-22 It is well known that degree of crystallinity influences on properties of polymers, and XRD is widely used for analysis of the crystallinity of polymeric materials.
Length of the ethylene sequence of EVA is restricted by positions of the VA units as shown in Scheme 2. Paraffin wax has stacked lamella crystals due to relatively short methylene sequence, while polyethylene (PE) has chain-folding crystalline structures due to long methylene sequence.23-27 EVA should have stacked lamella crystals like paraffin wax because of relatively small ethylene block size. In general, EVAs have two crystalline structures of [110] and [200]. Crystalline structure and ethylene block size of an EVA should be influenced not only by the VA content but also by positions of the VA units in an EVA chain. Thus, crystalline structures and ethylene block sizes of EVAs with the same VA content may be different each other if the distribution patterns (or uniformities) of the VA units are different as described in Scheme 2.
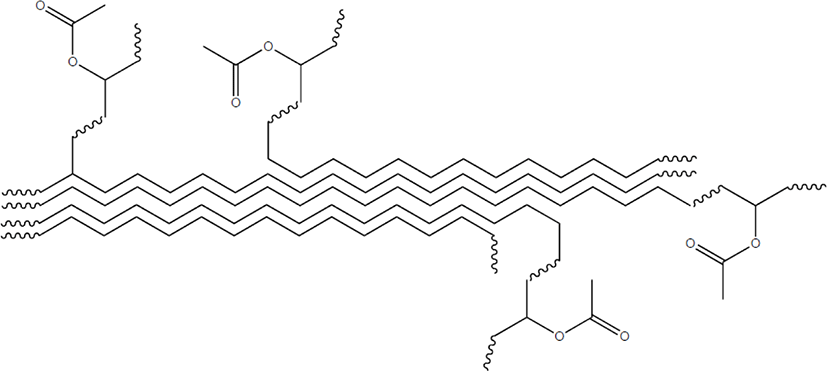
In this study, crystalline properties of various EVAs with the VA contents of 3.3-17.8 mol% (9.5-40 wt%) were analyzed using XRD and influence of microstructures of the EVAs on the crystalline properties was investigated. Percentage crystallinity (Xc), interplanar crystal spacing (dhkl), and crystal stack size (Dhkl) were compared as the crystalline properties. Three sets of EVAs having the same VA contents were employed to examine the influence of microstructures on the crystalline properties in detail. Relative superiority for the percentage crystallinity and the number of crystal plane piles (Nhkl) of [110] and [200] crystals was also investigated.
Experimental
Twelve EVAs with different VA contents of three suppliers were used: four EVAs of Aldrich Co. (USA) (marked to EVA 12, EVA 18, EVA 25, and EVA 40 with the VA contents of 12, 18, 25, and 40 wt%, respectively), four EVAs of DuPont Co. (USA) (EVA 770, EVA 450, EVA 360, and EVA 265 with the VA contents of 9.5, 18, 25, and 28 wt%, respectively), and four EVAs of Hanwha Co. (Republic of Korea) (EVA 1315, EVA 1316, EVA 1317, and EVA 1328 with the VA contents of 15, 18, 22, and 28 wt%, respectively). Weight percentages (wt%) of the VA contents were converted to the mole percentages (mol%) (Table 1). Three EVAs of EVA 18, EVA 450, and EVA 1316 have the same VA content of 6.7 mol%. Two sets of EVAs (EVA 25/EVA 360 and EVA 265/EVA 13128) have the same VA contents of 9.8 and 11.2 mol%, respectively.
EVA | Vinyl acetate content (mol%) |
---|---|
EVA 770 | 3.3 |
EVA 12 | 4.3 |
EVA 1315 | 5.4 |
EVA 18 | 6.7 |
EVA 450 | 6.7 |
EVA 1316 | 6.7 |
EVA 1317 | 8.4 |
EVA 25 | 9.8 |
EVA 360 | 9.8 |
EVA 265 | 11.2 |
EVA 1328 | 11.2 |
EVA 40 | 17.8 |
Crystalline properties of EVA samples were measured using XRD. XRD analysis was performed using a D-max 2500/PC diffractometer (Rigaku Co., Japan) with Cu-Kα radiation. The accelerating voltage and electric current were 40 kV and 100 mA, respectively. The samples were scanned from 10° to 35° at a scan speed of 2°/min. The degree of crystallinity determined by XRD was calculated as the percentage of the scattered intensity of the crystalline phase over the sum of the scattered intensities of the crystalline and amorphous phases. The percentage crystallinity was acquired from the peak at 12° to 28° using XRD analysis software (Mdi Jade 6.0).
The percentage crystallinity (Xc, %) was calculated using equation (1).
where Ic and Ia are the sums of the intensities of the crystalline and amorphous regions, respectively. The interplanar crystal spacing of (hkl) planes, dhkl was calculated according to Bragg’s law of equation (2).
where λ is the wavelength of the X-ray (Cu-Kα = 0.154 nm) and θ is the Bragg angle. The stack (or particle) size of crystal was calculated according to Scherrer formula of equation (3).
where Dhkl is the interfacial distance (perpendicular to the plane of the crystal particle), K is the Scherrer constant (0.89 is selected), λ is the wavelength of the X-ray, B1/2 is the FWHM (radian), and θ is the Bragg angle.
Results and Discussion
Raw XRD pattern was smoothed for following deconvolution process to separate the crystalline and amorphous regions. The smoothed curve was deconvoluted to one amorphous and two crystalline peaks as shown in Figure 1. Two crystalline peaks at 2θ = 21.4 and 23.7° corresponding to [110] and [200] crystals, respectively, were observed. Full width at half maximum (FWHM) of the crystalline peak was also measured. The Bragg angles (2θ) and FWHMs of EVAs were summarized in Table 2. Ranges of the Bragg angles of [110] and [200] peaks were 21.04-21.68 and 23.26-23.92°, respectively. Ranges of the FWHMs of [110] and [200] peaks were 0.76-1.67 and 0.99-2.29°, respectively. The FWHMs of [110] peaks were narrower than those of [200] ones. The FWHM of [110] peak tended to increase as the VA content increased.
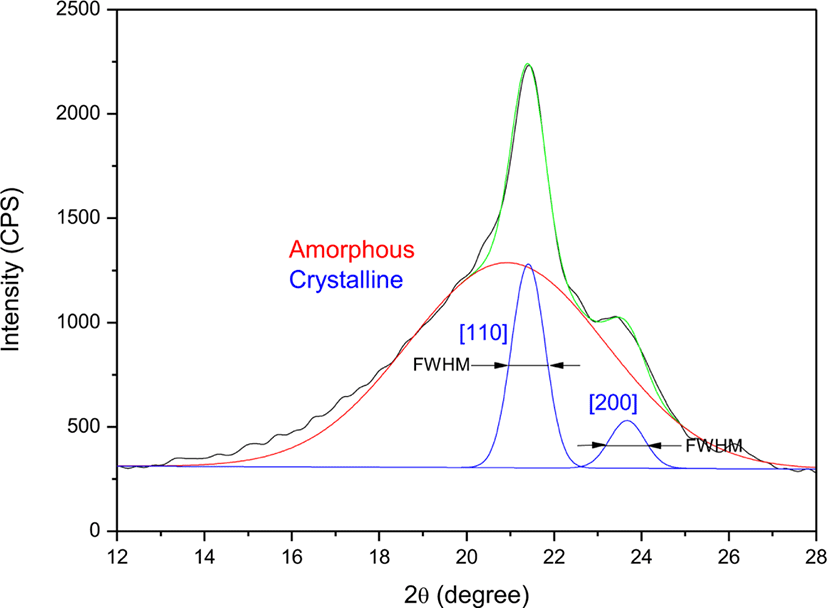
The Xc variation with the VA content was plotted as shown in Figure 2. The total Xc of [110] and [200] crystals decreased by about 3% every 1 mol% of the VA content. The individual Xcs of [110] and [200] crystals decreased by about 2.3 and 0.7%/mol%, respectively. After 10 mol% of the VA content, the decrement was very slight. There were some cases largely deviated from the fitted curve. For EVAs with 9.8 mol% of the VA content (EVA 25 and EVA 360), the Xcs of EVA 25 were lower than the fitted line but those of EVA 360 were much higher than the fitted line. If the VA units are evenly distributed, there will be few large ethylene blocks and crystalline structures may not be well developed. If the VA units of an EVA with 10.0 mol% of the VA content are absolutely evenly distributed, the ethylene block size is ‘9’ of ~VA-(CH2CH2)9-VA~. Difference in the melting points of paraffin wax and its ester is about 15°C, and the total number of ethylene units not to participate in crystal formation by the prevention of VA units in both sides is about ‘8’.28,29 Thus, the number of ethylene sequence to participate in the crystal formation is just ‘1’ for an EVA with the VA content of 10.0 mol% when the VA units are completely evenly distributed. Hence, it can be expected that distribution uniformity of the VA units of EVA 25 will be greater than that of EVA 360.
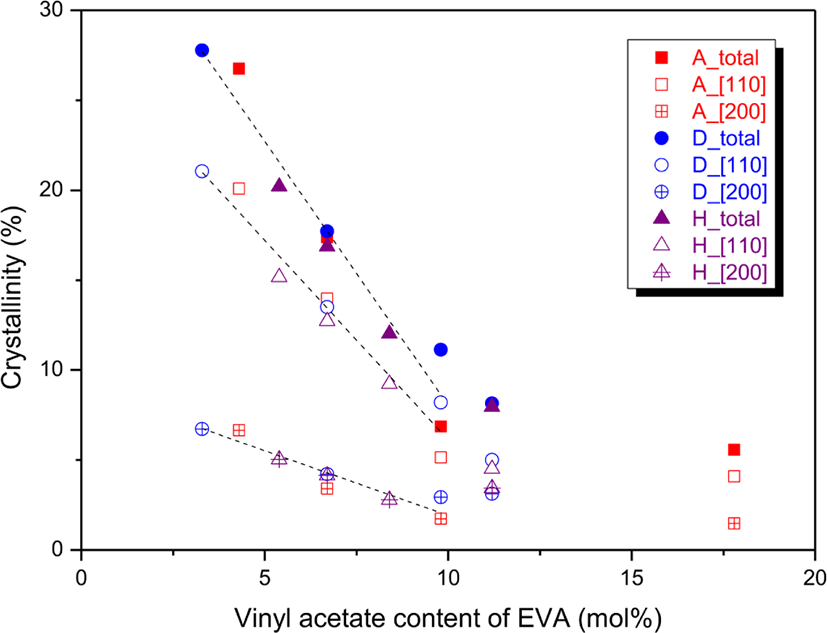
There are two crystalline structures of ethylene blocks of ~VA-(CH2CH2)n-VA~ (n > 20 and 15 < n < 20) in EVA.29 The large and small ethylene block sizes may belong to the [110] and [200] crystals, respectively. The Xc ratio of [110] and [200] crystals (Xc[110]/Xc[200]) was plotted as a function of the VA content as shown in Figure 3. The Xc ratio on the whole slightly decreased with increase in the VA content. It means that crystal formation of the big ethylene block was more decreased than that of the small one by increasing the VA content. Except for three EVAs (EVA 18, EVA 265, and EVA 1328), the Xc ratios were about 3. The Xc ratio of EVA 18 (6.7 mol% of the VA content) was larger than those of the other EVAs. This is because EVA 18 has higher [110] crystallinity as well as lower [200] one compared to the other EVAs with the same VA content as shown in Figure 2. This implies that there are longer ethylene sequences in EVA 18 due to more inhomogeneous distribution of the VA units. The Xc ratios of EVA 265 and EVA 1328 (their VA contents are the same of 11.2 mol%) were much lower than those of the other EVAs since they had relatively higher Xcs of the [200] crystal. Thus, EVA 265 and EVA 1328 should have more homogeneous distribution of VA units than the other EVAs.
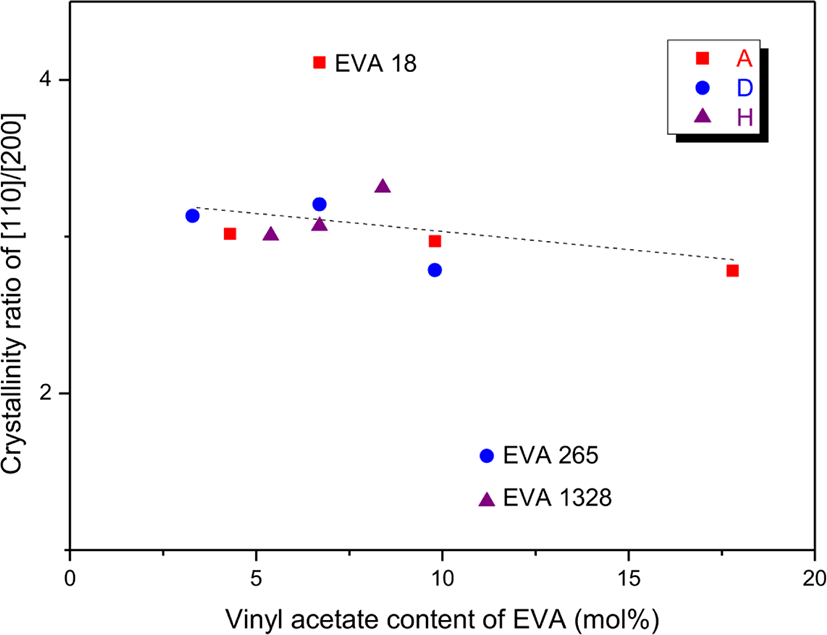
Variations of the dhkl and Dhkl were plotted as a function of the VA content as shown in Figures 4 and 5, respectively. The d110s and d200s were 0.410-0.422 and 0.372-0.382 nm, respectively (Figure 4). The d110s were greater than the d200s and the difference was about 0.04 nm. The d110 and d200 on the whole increased as the VA content increased, and the increment of d110 was slightly larger than that of d200. This means that distance between EVA chains becomes longer by prevention of the VA units. The D110s and D200s were 9.564-21.918 and 6.997-16.417 nm, respectively (Figure 5). The D110 was greater than the D200, and the difference became smaller by increasing the VA content. The D110 and D200 on the whole decreased with increase in the VA content, and the decrement of D110 was greater than that of D200. The decreased Dhkls can be explained by reduction of the number of ethylene sequences participating in the crystal formation. Some EVAs of the same supplier had similar Dhkls though their VA contents are very different. For instance, EVA 450, EVA 360, and EVA 265 (6.7-11.2 mol% of the VA content) had similar D110 of 17.32-17.09, and EVA 1315, EVA 1316, and EVA 1317 (5.4-8.4 mol% of the VA content) had similar D200 of 13.16-13.45. The Dhkls of EVAs with the same VA content showed big difference according to the suppliers. For EVA 18, EVA 450, and EVA 1316 with the same VA content of 6.7 mol%, the order of D110 was EVA 1316 > EVA 18 ~ EVA 450, whereas that of D200 was EVA 18 > EVA 450 > EVA 1316. For EVA 25 and EVA 360 with the VA content of 9.8 mol%, the D110 of EVA 360 was greater than that of EVA 25, whereas the D200 of EVA 360 was smaller than that of EVA 25. Thus, it can lead to a conclusion that the Dhkls are largely influenced by the VA content and the distribution uniformity of VA units.
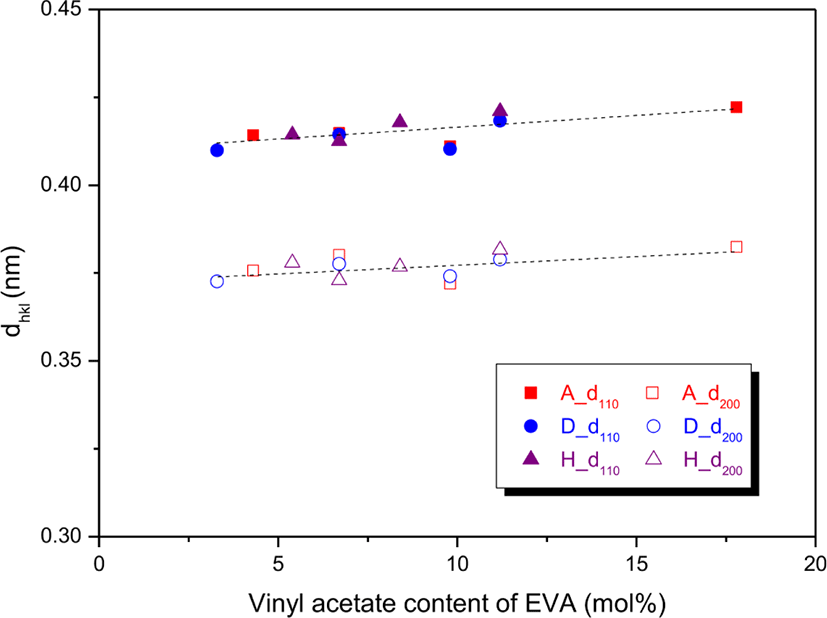
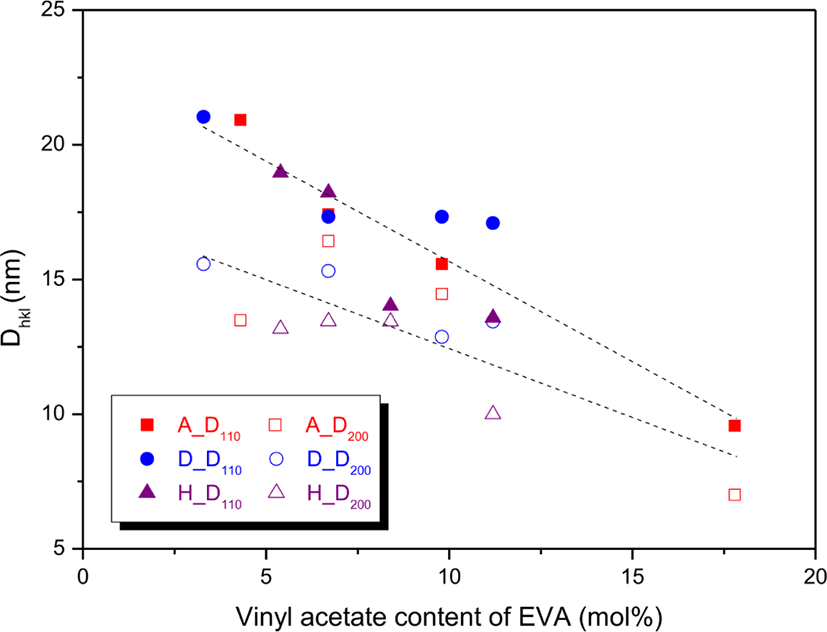
The number of crystal plane piles (Nhkl) was calculated using equation (4).30
The N110s and N200s were 22.7-51.3 and 18.3-43.2, respectively. Figure 6 shows variations of the N110 and N200 with the VA content. The N110 decreased with increase in the VA content by about twice every 1 mol% of the VA content, and it will be lower than 10 when the VA content is higher than 24.8 mol%. This implies that not only the ethylene block size but also the number of EVA chains participating in the [110] crystal formation decreased by increasing the VA content. The N200 did not show notable change until about 10 mol% of the VA content and then showed a decreased trend. The N110s were generally larger than the N200s, but EVA 18, EVA 25, and EVA 1317 showed the reverse trends. The larger N110s than N200s implies that the number of EVA chains with long ethylene sequences is greater than that of EVA chains with short ones. On the contrary to this, for EVA 18, EVA 25, and EVA 1317, the number of EVA chains with long ethylene sequences might be smaller than that of EVA chains with short ones.
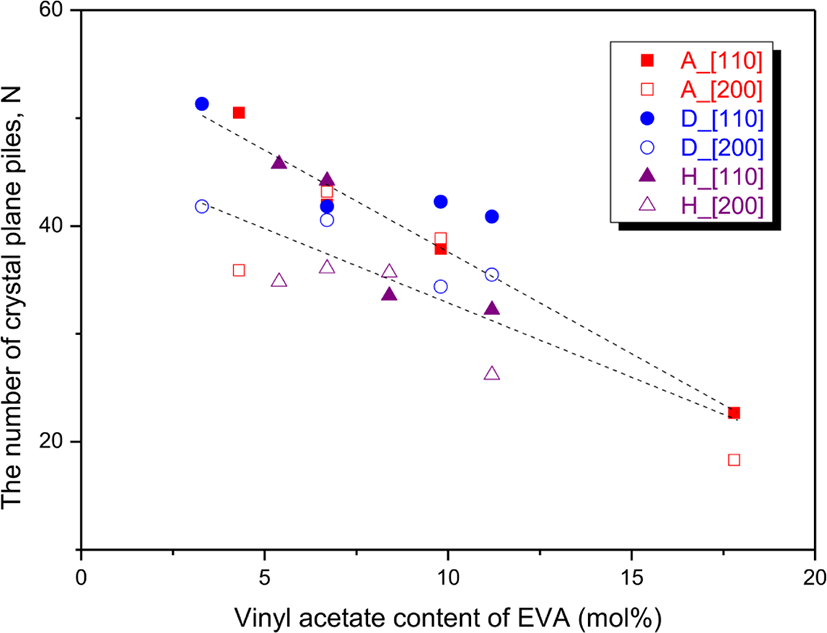
The N110s and N200s of EVAs with the same VA content also showed different trends according to the suppliers. For EVA 18, EVA 450, and EVA 1316 with the same VA content of 6.7 mol%, the N110 of EVA 1316 was greater than those of the others whereas the N200 of EVA 1316 was smaller than those of the others. And total Nhkls of the N110 and N200 were also different each other. The total Nhkls of EVA 18, EVA 450, and EVA 1316 were 85.2, 82.3, and 80.3, respectively. Flexibility and transparency of EVA with smaller Nhkls might be better than those of EVA with larger ones. For EVA 25 and EVA 360 with the same VA content of 9.8 mol%, the N110 of EVA 360 was greater than that of EVA 25 but the N200 of EVA 360 was smaller than that of EVA 25 though the total Nhkls were the same of 76.7 and 76.6 for EVA 25 and EVA 360, respectively. For EVA 265 and EVA 1328 with the same VA content of 11.2 mol%, both the N110 and N200 of EVA 265 were greater than those of EVA 1328. The total Nhkl of EVA 265 (76.3) was greater than that of EVA 1328 (58.5) by 1.3 times. This denotes that EVA 1328 would be more flexible and transparent than EVA 265.
Figure 7 shows variation of the N110/N200 ratio with the VA content. Except for some EVAs, the N110/N200 ratios were about 1.2. This implies that the number of EVA chains participating in [110] crystal formation is greater than that of EVA chains participating in [200] one by about 20%. EVA 12 had the greatest N110/N200 ratio of 1.41. The N110/N200 ratios of EVA 450, EVA 18, and EVA 25 were nearly 1.00 (1.03, 0.97, and 0.98, respectively). The N110/N200 ratio of EVA 1317 (0.94) was lower than the others. EVAs with the average low N110/N200 ratio might have the long ethylene sequences less than the short ones. Especially, EVA 1317, EVA 25, and EVA 18 would have the long ethylene sequences less than the short ones as discussed previously.
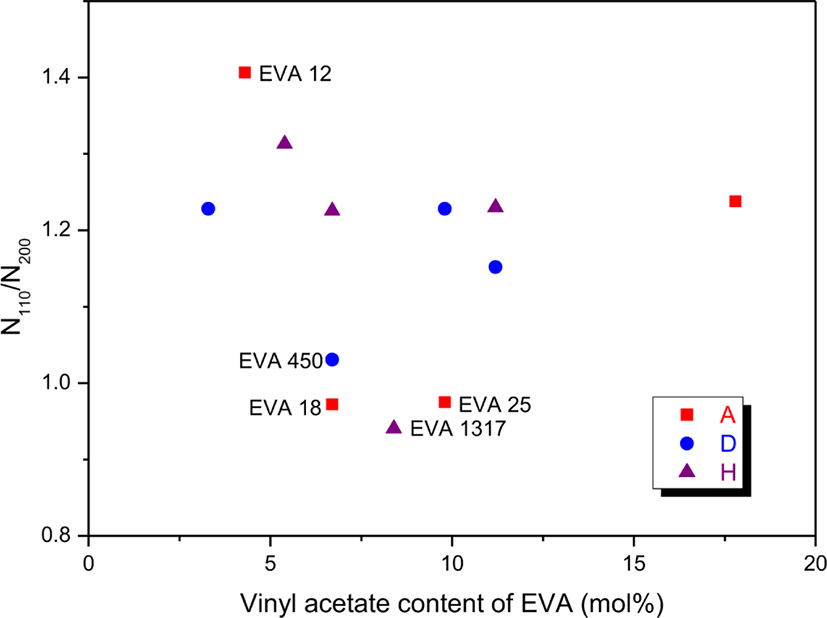
Influence of the VA content and distribution uniformity of EVA on the crystalline properties was summarized in Table 3. The total Xc notably decreased by increasing the VA content, while it slightly decreased by increasing the distribution uniformity. The Xc[110]/Xc[200] ratio was more affected by the distribution uniformity than the VA content. The d110 and d200 slightly increased by increasing the VA content, but the distribution uniformity did not affect the d110 and d200. The D110 and D200 decreased by increasing the VA content, whereas the D200 slightly increased by increasing the distribution uniformity. Variations of the N110 and N200 according to the VA content and distribution uniformity showed similar trends to those of the D110 and D200. The N110/N200 ratio slightly decreased by increasing the distribution uniformity, but the VA content did not affect the N110/N200 ratio.
Crystalline property | VA content | Distribution uniformity |
---|---|---|
Total crystallinity | ↓↓↓ | ↓ |
Xc[110]/Xc[200] | ↓ | ↓↓ |
d110 | ↑ | --- |
d200 | ↑ | --- |
D110 | ↓↓ | ↓ |
D200 | ↓ | ↑ |
N110 | ↓ | ↓ |
N200 | ↓ | ↑ |
N110/N200 | --- | ↓ |
Conclusions
Two crystalline peaks at 2θ = 21.0-21.7° and 23.2-24.0° of the [110] and [200] crystals of EVA were corresponded to the large and small ethylene block sizes, respectively. The Xc ratios of [110] and [200] were about 3 except for some EVAs, and it on the whole slightly decreased with increase in the VA content of EVA. The total Xc decreased by about 3%/mol%, while the individual Xcs of [110] and [200] decreased by about 2.3 and 0.7%/mol%, respectively. Formation of the crystalline structures of EVA became more difficult by increasing the distribution uniformity. The d110s and d200s were 0.410-0.422 and 0.372-0.382 nm, respectively, and they on the whole increased as the VA content increased. The D110s and D200s were 9.564-21.918 and 6.997-16.417 nm, respectively, and they on the whole decreased with increase in the VA content. The N110s and N200s were 22.7-51.3 and 18.3-43.2, respectively, and the N110 decreased with increase in the VA content by about 2/mol%. The N110/N200 ratios were about 1.2 except for some EVAs. For some EVAs, the crystalline properties were largely deviated from the average trends. EVAs with the same VA content of different suppliers showed some different crystalline properties. These were explained by difference in their microstructures, especially degree of the distribution uniformity. Difference in the crystalline properties due to the microstructures could influence on properties of EVAs such as flexibility and transparency.