Introduction
최근 전 세계적으로 미세먼지 및 대기오염 악화에 대한 이슈가 커져가고 있다. 인구와 자동차 및 도로가 집중되어 있는 대도시의 경우 증가하는 자동차와 도로 확장으로 인해 미세먼지에 의한 대기오염이 더욱 심각해지고 있으며, 자동차의 직간접적인 영향이 커 그에 대한 대책 및 해결 방안을 필요로 하고 있다.1 과거에는 차량의 배출가스가 미세먼지 발생 기여도에서 큰 비중을 차지하였다. 하지만 근래에는 자동차 배출가스 규제의 강화로 인해 차량에서 배출되는 배출 미세먼지(Exhaust PM)는 감소하고, 반대로 타이어/브레이크 마모, 도로 파손 등에 의해 발생하는 비-배출 미세먼지(Non-exhaust PM)의 배출량은 상대적으로 증가하는 추세이다.2-4 특히, 타이어 마모입자는 대기오염 및 인체 건강에 영향을 미칠 수 있는 인자로 파악되고 있어 타이어 마모에 직접적인 영향을 주는 트레드의 개선이 필요한 상황이다.5 현재까지 타이어에서 발생하는 미세먼지 저감 방안은 타이어의 내마모성능을 증가시키는 것으로 보고되었다.6-9 하지만 이전 연구에서는 상용화된 타이어 제품을 사용해 다양한 주행 조건에서의 평가만 이루어졌고, 아직까지 고무 컴파운드의 조성에 따른 미세먼지 발생에 관한 연구는 이루어지지 않았다.6-9
일반 자동차용 타이어는 크게 passenger car radial (PCR) 타이어, light truck radial (LTR) 타이어, truck bus radial (TBR) 타이어로 구분되며, 그 중 TBR 타이어의 경우 타이어 마모로 인한 미세먼지 배출량의 40% 이상을 점유하고 있다.10 또한 최근 TBR 타이어는 전기차 및 자율주행 기술의 개발 등으로 인해 타이어에 우수한 연비성능 및 내마모성능이 요구되고 있다.11,12
TBR 타이어 트레드는 강도가 우수하고 내 cut & chip 성능이 우수한 natural rubber (NR)를 base rubber로 사용하며, 내마모성능을 위해서 high cis- butadiene rubber (BR)를 blend 하여 사용한다. 또한 TBR 타이어 트레드는 NR을 base rubber로 사용함에 따라 NR과 상용성이 뛰어난 카본블랙을 주로 보강제로 사용해왔다. 하지만, 최근에는 연비성능 개선을 위해 카본블랙 일부를 대체하여 실리카를 사용한 연구가 이루어지고 있다.13-15 실리카는 카본블랙보다 우수한 연비성능을 나타내지만 TBR 타이어 트레드의 경우NR을 base rubber 로 사용함에 따라 NR에 포함된 단백질과 인지질 및 NR의 큰 분자구조로 인해 실리카의 실란화반응과 실란커플링제와 고무의 커플링반응이 쉽게 일어나지 않아 카본블랙보다 불리한 내마모성능을 나타내는 문제점을 가지고 있다.16,17
이러한 문제점은 NR에 실리카 친화형 관능기인 epoxide 기를 도입하여 해결될 수 있다.17-19 최근 silica filled ENR 컴파운드는 실리카의 hydroxyl 기와 ENR의 epoxide 기 사이의 화학적 결합을 통해 filler-rubber interaction을 향상시키며, 불리한 내마모성능을 개선할 수 있다고 보고된 바 있다.20,21
따라서 본 연구에서는 실리카 친화형 관능기를 가지는 ENR을 이용하여 binary filler 가 적용된 ENR/BR blend 컴파운드에서 카본블랙과 실리카의 비율 변화에 따른 컴파운드의 내마모성능 및 연비성능의 변화를 NR/BR blend 컴파운드와 비교하여 평가하였다. 또한, 컴파운드에서 발생하는 미세먼지 발생량을 측정하여 미세먼지 발생량이 적은 TBR 타이어 트레드 컴파운드 개발의 guide line을 제시하고자 한다.
Materials and Experimental Methods
본 실험에서는 base rubber 로 NR (Standard Vietnamese Rubber SVR-10, dirty contents 0.1 wt%), ENR-25, ENR-50 (Epoxyprene 25, Epoxyprene 50, Muang Mai Guthrie Public Co., Ltd., Muang Phucket, Thailand)을 사용하였으며, BR (NdBR-60, Kumho Petrochemical Co., Daejeon, Korea)을 blend 하여 사용하였다. Silica (Ultrasil 7000 GR, Evonik Industries AG, Essen, Germany, Brunauer-Emmett-Teller (BET) surface area: 160-175 m2/g)와 carbon black (N234, OCI Company Ltd, Seoul, Korea)을 binary filler 로 사용하였으며, bis-[3-(triethoxysilyl)propyl]-tetrasulfide (TESPT, Si-69, Evonik Korea Ltd., Korea)가 silane coupling agent 로 사용되었다. Activator로 ZnO와 stearic acid (both from Sigma-Aldrich Corp., Seoul, Korea), 산화방지제로 N-(1,3-dimethyl butyl)-N′-phenyl-p-phenylenediamine (6PPD, Kumho Petrochemical Co., Daejeon, Korea)이 compounds에 사용되었다. 그리고 crosslinking agent 로 sulfur (Daejung Chemicals & Metals Co., Siheung, Korea), cure accelerator로 N-tert-butyl-2-benzothiazyl sulfonamide (TBBS, Shandong Yanggu Huatai Chemical Co., Ltd., Liaocheng, China)와 1,3-diphenylguanidine (DPG, 98%, Tokyo Chemical Industry Co. Ltd., Tokyo, Japan), pre vulcanization inhibitor로 N-cyclohexylthio phthalimide (PVI, Shandong Yanggu Huatai Chemical Co., Ltd., Liaocheng, China) 가 사용되었다.
ENR의 epoxide contents 측정을 위해 proton nuclear magnetic resonance spectroscopy (1H NMR; Varian, Unity Plus 300 spectrometer, Garden State Scientific, Morristown, NJ, USA)를 사용하였다. ENR samples은 deuterochloroform (CDCl3, Cambridge Isotope Laboratories, Inc., Andover, MA, USA)을 용매로 하여 5 mm NMR tube에 15 mg/mL 농도로 용해시켰다.
NR 및 ENR의 glass transition temperature (Tg)를 differential scanning calorimeter (DSC-Q10, TA instruments, USA)를 이용해 측정하였다. Rubber sample (3-6 mg)을 heating rate 10°C/min를 적용하여 -80°C에서 100°C까지 측정하였다.
컴파운드의 무니점도는Mooney viscometer (Vluchem IND Co., Seoul, Korea)를 이용해 측정하였다. Rotor의 회전속도는 2 rpm, 온도는 100°C로 설정하고 1분간의 예열 이후 4분간 rotor를 회전시켜 torque를 측정하였다.
Bound rubber contents 측정을 위해1st stage mixing 후의 컴파운드 0.2±0.01g을 filter paper에 넣고 25°C에서 6일 동안 toluene 100ml에 침지시켜 unbound rubber를 추출하였다. 그 뒤, toluene을 acetone으로 세척한 뒤 건조하였다. Bound rubber contents는 실험 전후 무게를 측정하여 계산하였다.
wfg: weight of the filler and gel
wt: weight of the specimen
mf: weight fraction of the filler in the compounds
mr: weight fraction of the polymer in the compounds
컴파운드의 cure characteristics은 moving die rheometer (MDR, Myung Ji Co., Seoul, Korea)을 이용하여 진동 각도 ±1°, 온도 150°C를 유지한 상태에서 30분 동안 작동하였으며, minimum torque (Tmin), maximum torque (Tmax), scorch time (t10) 그리고 optimal cure time (t90)을 측정하였다.
가교밀도는 가황된 시편을 10 mm × 10 mm × 2 mm로 준비해 tetrahydrofuran (THF, 99%, Samchun Chemical Co., Seoul, Korea) 과 n-Hexane (95%, Samchun Chemical Co., Seoul, Korea)에 각각 25°C에서 1일간 침지시켜 시편 내부의 유기 첨가물을 제거하였다. 그 후, 시편의 무게를 측정한 후 상온에서 1일간 톨루엔 용매에서 침지시키고 팽윤된 시편의 무게를 측정하여, Flory-Rehner equation 식을 통해 가교밀도를 계산하였다.
ν: Crosslink density (mol/g)
Mc: Average molecular weight between crosslink points (g/mol)
Vr: The volume fraction of rubber in the swollen gel at equilibrium
Vs: The molar volume of solvent(cm3/mol)
ρr: The density of the rubber sample (g/cm3)
χ: The polymer-solvent interaction parameter
기계적 특성 확인을 위해 universal testing machine (UTM, KSU-05M-C, KSU Co., Ansan, Korea)을 이용하여 ATSM D 412에 따라 제작된 100 mm (길이) × 25 mm (너비)의 아령형 시편을 500 mm/min의 속도로 측정하였다.
내마모성능은 Deutsche Industrie Normen (DIN) 마모시험기를 이용하여 DIN 53516에 따라 측정하였다. 시편은 직경 16 mm, 두께 8 mm인 실린더형으로 제작하였고, 40±1 rpm으로 회전하고 있는 원통형 드럼에 부착된 abrasive sheet 표면에 5 N의 load를 가한 시편을 40 m 주행시키고 감소된 질량을 측정하였다.
Vulcanizates의 점탄성 특성을 확인하기 위해 dynamic mechanical thermal spectrometer (DMTS, EPLEXOR 500N, GABO, Ahlden, Germany)를 이용해 온도 60°C, 10 Hz frequency에서 strain을 0.5%부터 10%까지 strain sweep 하여 tan ẟ를 측정하였고, dynamic mechanical analyzer (DMA Q800, TA Instrument, USA)를 이용하여 strain 0.2%, 10 Hz frequency에서 -80°C 부터 100°C까지 temperature sweep 하여 storage modulus (E′), loss modulus (E″), tan ẟ를 측정하였다.
타이어 트레드 시편의 미세먼지 측정은 트레드 시편을 마모시키면서 aerodynamic particle sizer (APS, TSI 3321, USA)를 사용해 미세먼지의 질량, 수 농도 및 입경분포도를 측정하였다. 시험의 개략도는 Figure 1과 2에 나타내었다. 마모시험기를 사용하여 시편 마모시에 발생하는 미세먼지를 측정하였다. 시편과 사포 벨트를 접촉시킨 상태에서 시편에 수직방향으로 20 N의 하중을 가하고, 사포 벨트를 20 m/min의 속도로 회전시켜 시편을 마모시켰다. 사포 벨트는 입도 80을 사용하였다. 본 연구에서는 에어필터(filtered air supply, TSI 3074B, USA)와 울파필터(ULPA)를 이용하여 수분 및 입자가 없는 깨끗하고 건조한 공기를 유량계 (flow meter, TSI 5300, USA)로 측정하여 일정 유량 (5 L/min)으로 챔버 내로 공급하였다. 최종적으로 시편의 마모 시 발생하는 미세먼지를APS를 이용하여 유량 1 L/min으로 0.37~20 μm 범위의 입자를 측정하였다.
컴파운드 배합표는 Table 1에 나타내었다. 배합표를 바탕으로 밀폐식 혼합기(300cc, Mirae Scientific Instruments Inc., Gwangju, Korea)를 이용하여 컴파운드를 제조하였다. Fill factor는 혼합기 용량의 80%로 설정하였으며, 투입 단위는 parts per hundred rubber (phr) 로 rubber를 기준으로 투여되었다. 배합 절차는 Table 2에 나타내었고, 각 stage의 initial temperature는 100°C와 50°C, dump temperature는 150~155°C 와 80~90°C로 control 하였다. 각 stage의 mixing 이 끝난 후에는 two-roll mill을 이용하여 컴파운드를 sheet 화 하였다. 이렇게 제조한 컴파운드는 MDR을 이용하여 150°C에서 optimal cure time을 확인한 후 150°C의 유압프레스에서 최적 가황 시간동안 가류하여 가황물을 제조하였다.
Results and Discussion
Base rubber로 사용한 NR, ENR-25, ENR-50의 1H NMR spectrum은 Figure 3에 나타냈고, epoxide contents 및 Tg는 Table 3에 나타내었다. 1H NMR spectrum은 5.1 ppm에서 olefinic methine protons에 의해 모든 rubber에서 signals이 나타났고, 2.7 ppm에서는 epoxy methine protons에 의해 ENR-25, ENR-50에서만 signals이 나타났다. 3.6 ppm에서는 adjacent epoxide groups의 ring opening으로 생성된 five cyclic ether 구조로 인한 signals이 나타났다.22 5.1 ppm과 2.7 ppm에서의 두 peak 면적을 이용해 epoxide contents를 계산하였다.23,24 NR, ENR-25, ENR-50의 Tg는 DSC를 통해 측정하였고 epoxide contents가 증가함에 따라 chain mobility가 제한되어 rubber의 Tg가 증가하였다.23,25 ENR의 Tg는 epoxide contents 1 mol% 당 0.75~0.81°C 정도 증가하였다.
Rubber | 1H NMR results | DSC results |
---|---|---|
Epoxide contents (%) | Tg (°C) | |
NR | 0 | -61.5 |
ENR-25 | 25.3 | -42.2 |
ENR-50 | 46.2 | -25.2 |
Mooney viscosity 및 bound rubber 측정 결과와 moving die rheometer (MDR)를 이용해 측정한 cure characteristics 결과를 Figure 4, Table 4에 나타내었다. CB/Silica 비율이 35/20인 컴파운드에서 NR/BR blend 컴파운드는 NR의 큰 분자량과 단백질과 인지질로 인해 형성된 NR의 network로 인해 ENR/BR blend 컴파운드보다 높은 무니점도와 높은 bound rubber 함량을 나타냈다.26,27 CB의 함량이 감소할수록 NR/BR blend 컴파운드는 CB-rubber interaction의 감소로 낮은 bound rubber 함량을 나타냈고, 반면에 ENR/BR blend 컴파운드는 실리카 친화형 관능기인 epoxide group에 의해 실리카 함량이 증가함에 따라 silica-ENR interaction 증가로 bound rubber 함량이 증가하였으며, 그로 인해 무니점도가 증가하는 결과를 나타냈다.28 Cure characteristic은 실리카 비율이 증가함에 따라 실리카의 촉진제 흡착으로 인해 t90이 증가하는 결과를 나타내었고, epoxide group 이 많을수록 base rubber의 이중결합 수 감소로 인해 t90이 늘어나는 결과를 나타냈다. 또한 ENR/BR blend 컴파운드는 150°C 조건에서 silica-ENR interaction을 형성함에 따라 epoxide group이 많을수록 높은 ∆T 값을 나타내었다.
Mechanical properties 결과는 Figure 5와 Table 5에 나타내었고, crosslink density 결과는 Table 5에 나타내었다. NR/BR blend 컴파운드는 실리카 비율이 증가함에 따라 실리카의 촉진제 흡착과 CB-rubber interaction의 감소로 인해 낮은 가교밀도를 나타내었다. ENR-25/BR blend 컴파운드는 silica-ENR-25 interaction에 의해 실리카 증량 시 가교밀도의 감소가 NR/BR blend 컴파운드에 비해 작았으며, ENR-50/BR blend 컴파운드는 높은 silica-ENR-50 interaction에 의해 실리카 증량에 따라 가교밀도가 증가되는 결과를 나타내었다.20 컴파운드의 modulus는 가교밀도와 filler-rubber interaction에 영향을 받는데, silica-rubber interaction에 의한 modulus 향상보다 CB-rubber interaction에 의해 향상되는 modulus가 높다고 보고되었다.29 따라서 실리카 비율 증가에 따라 낮은 가교밀도를 나타낸 NR/BR blend 컴파운드와 ENR-25/BR blend 컴파운드는 실리카 비율 증가 시 낮은 modulus를 나타내었고, ENR-50/BR blend 컴파운드는 실리카 비율 증가에 따라 높은 가교밀도를 나타내었음에도 비슷한 modulus를 나타냈다.
DIN abrasion test를 통해 컴파운드의 내마모성능을 평가하였고, 평가 결과는 Table 5에 나타냈다. 평가 결과 NR/BR blend 컴파운드는 실리카의 비율이 증가함에 따라 감소된 CB-rubber interaction에 의해 불리한 내마모성능을 나타내었고, 반면에 ENR/BR blend 컴파운드는 실리카 비율 증가에 따라 향상된 silica-ENR interaction으로 인해 내마모성능이 개선되는 결과를 나타냈다. 또한 epoxide group이 많을수록 높은 silica-ENR interaction을 가지므로 ENR-50/BR blend 컴파운드에서 가장 우수한 내마모성능을 나타냈다.
Dynamic viscoelastic properties에서 tan δ at 60°C 값은 tire의 rolling resistance (RR)를 나타내는 지표로서, 그 값이 낮을수록 fuel efficiency가 향상된다고 알려져 있다.30 Loss modulus (E″) at 0°C 값은 tire의 wet grip 성능을 나타내는 지표이며, 그 값이 높을수록 wet grip 성능이 우수해진다고 알려져 있다.31,32
Figure 6과 Table 6에 컴파운드의 dynamic viscoelastic properties 결과를 나타내었다. 고무 컴파운드는 Tg 부근에서 rubber chain의 hysteresis 로 인해 높은 tan δ 값을 나타낸다.33 또한 일반적으로 연비성능의 지표인 tan δ at 60°C 값은 rubber의 hysteresis 보다 filler의 재응집 및 파괴에 의한 hysteresis의 영향을 크게 받는다. 따라서 고무와 화학적 결합을 하는 ssilica와 SCA는 carbon black에 비해 변형에 의한 hysteresis를 줄일 수 있어 우수한 연비성능을 나타내는 것으로 알려져 있다.33 따라서 모든 컴파운드에서 실리카의 비율이 증가할수록 tan δ at 60°C 값이 낮아지는 것을 확인하였다. 하지만 ENR/BR blend 컴파운드는 epoxide contents가 증가함에 따라 높은 Tg를 나타내 컴파운드의 tan δ peak이 NR/BR blend 컴파운드에 비해 높게 나타나는 것을 확인하였다. 그에 따라 ENR은 epoxide contents가 증가할수록 높아진 Tg의 영향으로 tan δ at 60°C 값이 높게 나타나 불리한 fuel efficiency를 나타내었다. 반면 wet grip 성능의 지표인 E″ at 0°C 값은 높아져 epoxide contents가 증가됨에 따라 우수한 wet grip 성능을 나타내었다.
Figure 7과 Table 7에 컴파운드 마모 시 발생하는 미세먼지 측정결과를 나타냈다. 미세먼지 발생량은 내마모성능과 유사한 경향이 나타났다. NR/BR blend 컴파운드는 실리카 비율 증가에 따라 감소된 CB-rubber interaction으로 인해 불리한 내마모성능과 더불어 미세먼지 발생량 또한 증가한것으로 판단된다. 반면 ENR/BR blend 컴파운드는 실리카 비율 증가에 따라 개선된 내마모성능을 나타내었고, silica와 ENR이 chemical interaction을 통한 강한 filler-rubber interaction을 형성하기 때문에 미세먼지 발생량이 감소된것으로 판단된다. 결과적으로 강한 filler-rubber interaction을 가지는 silica filled ENR-50 컴파운드에서 가장 낮은 미세먼지 발생량을 나타냈다.
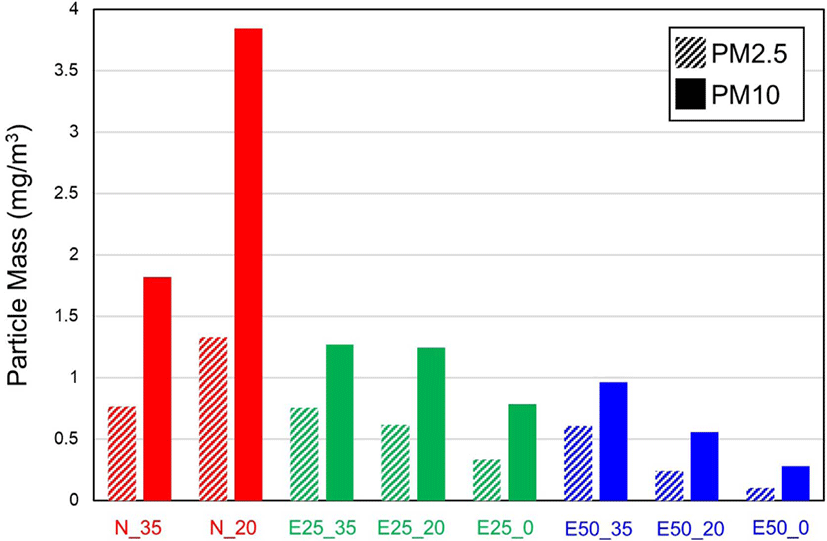
Conclusions
TBR tire tread 컴파운드는 우수한 내마모성능을 위해 BR을 blend 하여 사용하며, 최근에는 우수한 fuel efficiency가 요구됨에 따라 carbon black 일부를 silica로 대체하려는 연구가 이뤄지고 있다. 하지만 NR 컴파운드에서 카본블랙 일부를 실리카로 대체하면 연비성능은 개선이 되지만 내마모성능을 불리하다는 단점이 있다. 그에 따라 본 연구에서는 base rubber 로 NR이 아니라 실리카 친화형 관능기를 가지는 ENR-25, ENR-50을 사용하여 binary filler system에서 실리카 비율에 따른 컴파운드 물성을 비교하였다.
컴파운드 마모 평가 결과, NR/BR blend 컴파운드는 실리카 비율 증가 시 CB-rubber interaction의 감소로 낮은 bound rubber를 나타냈고, 낮아진 filler-rubber interaction으로 내마모성능이 불리하게 나타났다. 반면 ENR/BR blend는 실리카 비율 증가 시 silica-ENR interaction으로 인해 높은 bound rubber 와 filler-rubber interaction의 향상으로 내마모성능이 개선됨을 확인하였다. 따라서 epoxide group 함량이 높은 ENR-50/BR blend 컴파운드에서 높은 filler-rubber interaction으로 인해 가장 우수한 내마모성능을 나타내었다.
동적점탄특성에서 실리카 비율 증가 시 모든 컴파운드에서 연비성능이 개선됨을 확인하였고, epoxide group의 함량이 높을수록 base rubber의 Tg 증가로 인해 tan δ at 60°C 값이 증가해 불리한 fuel efficiency을 나타냈다. 하지만 Tg 상승으로 인해 E″ at 0°C 값은 높게 나타나 우수한 wet grip 성능을 나타냄을 확인하였다.
미세먼지 발생량은 컴파운드의 filler-rubber interaction에 따라 나타난 것으로 판단된다. 그에 따라 ENR/BR blend 컴파운드는 ENR이 carbon black 보다 실리카가 강한 interaction을 형성하기 때문에 실리카 비율 증가에 따라 미세먼지 발생량이 줄어들었다.
본 연구에서는 NR의 epoxidation이 silica filled 컴파운드의 filler-rubber interaction 향상으로 내마모성능의 개선에는 매우 효과적인 것을 확인하였다. 하지만 높은 epoxide contents는 컴파운드의 Tg 상승으로 인해 불리한 연비성능을 나타내었다. 또한 NR의 epoxidation으로 인한 filler-rubber interaction의 향상은 미세먼지 발생량을 감소시키는 것으로 판단된다. 결과적으로 ENR-25/BR blend 컴파운드는 ENR-50/BR blend 컴파운드 만큼 연비성능이 불리하지 않았으며, 실리카 비율이 증가함에 따라 연비성능, 내마모성능, 미세먼지 발생량이 개선되었다. 따라서 silica filled ENR-25/BR blend 컴파운드는 기존의 NR/BR binary filler system 컴파운드 보다 우수한 내마모성능, wet grip, fuel efficiency, 낮은 미세먼지 발생량을 나타내 보다 친환경적인 TBR tire tread의 개발이 가능하다. 본 연구 결과는 TBR tire tread 컴파운드의 물성에 epoxidation이 미치는 영향과 미세먼지 발생에 대한 중요한 지침으로 활용될 수 있을 것이다.