Introduction
In recent decades, water pollution has become not only a major threat to human survival and security but also a major obstacle to human health, economic and social sustainable development. Organic dyes have become one of the main pollu-tion sources of wastewater because of their extensive use in leather, textile, foodstuff, paper and other industries.1-4 Industrial organic wastewater containing complex components exhibit various undesirable properties to human environment, such as toxicity, chemical oxygen demand (COD), carcinogenicity, mutagenesis.5,6 Therefore, many studies of organic dyes treatment in wastewater are performed to reduce the pollution by industrial wastewater and protect the human ecological environment.
Various conventional biological and physicochemical methods including adsorption, chemical oxidation, and electrochemical treatment have been used for wastewater treatment.7-9 In recent years, a new advanced oxidation process (AOP) using active free radicals to degrade organic pollutants in wastewater is attracting attention of researchers.10,11 Irradiating ultraviolet light to H2O, CO2 and inorganic salts generates reactive free radicals (·OH). The photocatalytic degradation technology using the radicals is considered as an effective oxidation process for treatment of wastewater without secondary contamination.
Unique electronic and optical properties of group II-VI semiconductor materials like ZnS,12 CdS,13 CdSe,14 HgTe15 depend on their size and morphology. Among the semiconductors, cadmium selenide (CdSe) has been used as biological labels, biomedical imaging, photocatalysts, solar cells, and lasers due to its photoluminescence, electronic and optical performances.16-18 In the photocatalytic reaction, light absorption, separation and transmission of photogenerated electrons and holes, and the surface photochemical reaction are the three major influencing factors of photocatalytic activity.19 For CdSe, the extremely narrow band gap (about 1.7 eV) expanded the light adsorption range to visible light, while the rapid recombination of photogenerated electrons and holes reduced its photocatalytic performance. Many researchers have attempted to find a method to decrease the recombination rate of photogenerated electrons and holes.20,21 Doping with transition metal ions was regarded as an efficient method for suppressing the photogenerated electron-hole recombination.10,22,23 In this system, transition metal ions act as electron trapping sites, which can enhance the photocatalytic activity by trapping the photogenerated electrons in solution. Addition of carbon materials is also an important route to increase the photocatalytic activity of semiconductors.24-26 Carbon materials with a large surface area act as adsorbents in the reaction to adsorb the target material and promote the reaction.
CdSe is prepared by several techniques such as, solgel method, hydrothermal method, microwave irradiation method, sonochemical method, solvothermal method and chemical injection method.27-31 In this study, CdSe nanoparticles were successfully synthesized using a simple microwave irradiation method. Mn2+ was doped onto CdSe nanoparticles and nanocomposites were formed by combining with fullerene (C60). The prepared products were characterized by X-ray diffraction (XRD), Raman spectroscopy and scanning electron microscopy (SEM). Photocatalytic performance was evaluated by the degradation of BG, MB, MO, and RhB under UV lamp of 254 nm.
Experimental
Selenium (Se, 99.99%), brilliant green (BG) and methyl orange (MO) were supplied by Sigma-Aldrich. Sodium sulfite (Na2SO3), cadmium sulfate octahydrate (3CdSO4·8H2O), ammonia solution (NH3·H2O, 28.0-30.0%), manganese (II) sulfate monohydrate (MnSO4·H2O), tetrahy-drofuran (THF), methylene blue (MB), and rhodamine B (RhB) were purchased from Samchun Chemicals (Korea). Fullerene (C60) was obtained from Tokyo Chemical Industry Co., Ltd. All chemicals were used without further purification.
The nanocomposites of CdSe-Mn were synthesized using microwave method. In a typical procedure, 4.1667 g of sodium sulfite and 0.1667 g of selenium powder were mixed with 50 ml of deionized water and refluxed for 1 h to prepare solution A. Then, 1.0000 g of cadmium sulfate octahydrate was dissolved in 8 ml of deionized water, and 12 ml of 3 M ammonia solution was added dropwise subsequently to prepare solution B. Solution C was prepared by dissolving 0.0355 g of manganese sulfate in 10 ml of deionized water. All the solutions were mixed at room temperature and treated by microwave irradiation for 5 min. CdSe-Mn nanocomposites were obtained by washing the resulting precipitation with deionized water. CdSe-Mn-C60 nanocomposites were prepared by calcination method using as-synthesized CdSe-Mn nanocomposites and fullerene (C60) powder. In our case, 100 mg CdSe-Mn nanocomposites and 50 mg fullerene (C60) were mixed in 10 ml of tetrahydrofuran for 30 min. Then, the mixture was placed in a hood to evaporate tetrahydrofuran and calcined at 700 °C for 2 h in an electric furnace (AJEON Heating Industry Co., Ltd.) under an inert argon atmosphere to synthesize CdSe-Mn-C60 nanocomposites.
XRD patterns were obtained by a powder X-ray diffraction analysis (Bruker, D8 Advance) with Cu Kα radiation (λ = 1.54178 Å). The crystal size and phase purity of as-synthesized products were investigated by XRD patterns. The morphological properties of CdSe-Mn nanocomposites and CdSe-Mn-C60 nanocomposites were measured using scanning electron microscopy (SEM, JEOL Ltd, JSM-6510) at an acceleration voltage of 15 kV. Raman spectroscopy (BWS465-532S) was performed to study the lattice vibration of the products. UV-vis absorption spectra were recorded by UV–vis spectrophotometer (Shimazu UV-1619PC) in the wavelength of 200-800 nm.
The photocatalytic activity of as-synthesized CdSe-Mn-C60 nanocomposites was investigated by the degradation of organic dyes such as BG, MB, MO and RhB under irradiation of 254 nm using a UV lamp (8 W, 77202 MARNE LA VALLEE-Cedex 1 FRANCE). Typically, 10 ml of organic dye solution with a certain concentration (absorption intensity at 1.0) was prepared and injected into a reactor. After 5 mg of CdSe-Mn-C60 nanocomposites were added, the suspension was stirred in a dark environment for 30 min to get an adsorption–desorption equilibrium between organic dye and photocatalysts. Then, the reactor was irradiated with 254 nm UV lamp and 3 ml of samples were used for analysis at time intervals of 10 min.
Results and Discussion
Figure 1 showed the XRD patterns of as-synthesized CdSe-Mn-C60 nanocomposites. The distinct diffraction peaks at 2θ values of 24.61°, 26.12°, 27.82°, 35.12°, 42.82°, 46.61°, 50.69°, 59.33°, 64.68°, 67.22°, 73.20°, and 78.04° indexed by the CdSe crystalline planes of (100), (002), (101), (102), (110), (103), (112), (203), (210), (211), (105), and (300) according to JCPDS card number of 00-002-0330.27 The appearance of peak at 34.38° can be explained by the existence of Mn, which corresponds to (200) plane.32 The characteristic peaks located at 20.84°, 22.45°, 31.18°, and 33.22° can be identified as (311), (222), (422), and (511) planes of C60, respectively.33 The XRD patterns indicated that CdSe-Mn-C60 nanocomposites with good crystallinity were synthesized successfully.
The SEM images of CdSe-Mn nanocomposites and CdSe-Mn-C60 nanocomposites presented in Figure 2 were used to observe the structure and morphology. CdSe-Mn nanocomposites showed spherical and uniform structure with a diameter of less than 500 nm (Figure 2a). In the heterostructure of CdSe-Mn-C60 nanocomposites, there was no morphological change of CdSe-Mn nanocomposites and fullerene (C60) displays flagstone-like structure (Figure 2b). The surface of fullerene (C60) was completely or partially covered by CdSe-Mn nanocomposites.
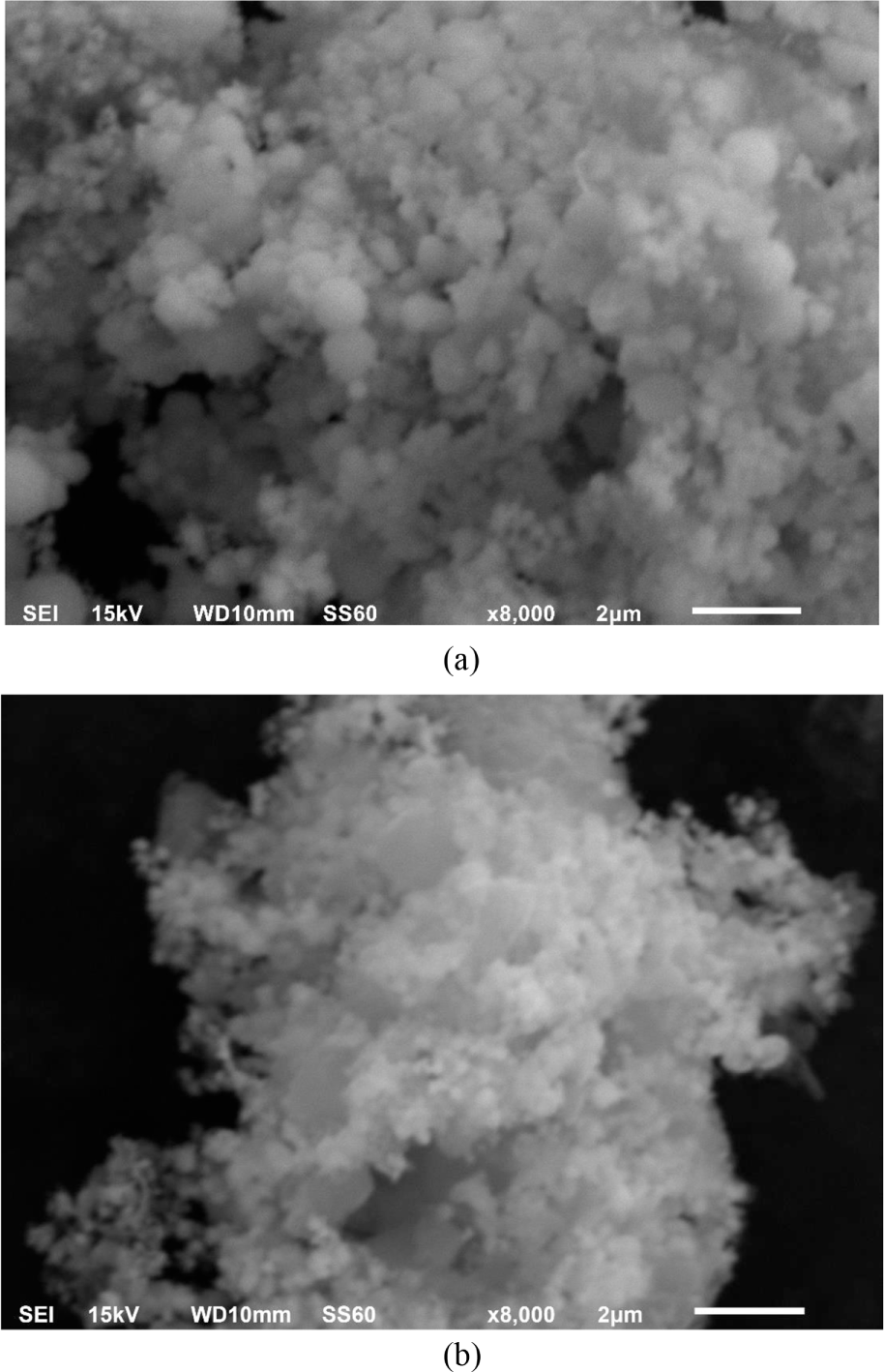
Figure 3a and 3b showed the Raman spectra of CdSe-Mn nanocomposites and CdSe-Mn-C60 nanocomposites obtained using a 532 nm laser at room temperature. Raman shifts of CdSe-Mn nanocomposites located at 202 cm-1, and 411 cm-1 corresponded to the l LO and 2 LO (longitudinal optical) vibration peaks of CdSe in Figure 3a.17 As shown in Figure 3b, the Ag (1) and Ag (2) vibrational modes of fullerene (C60) were observed at peaks of 491 cm-1 and 1460 cm-1. And the major bands at 269 cm-1 and 1577 cm-1, represented the Hg (1) and Hg (8) vibrational modes of fullerene (C60), respectively.34,35 Due to the high intensity of fullerene (C60), the bands of CdSe cannot be confirmed easily in the structure of CdSe-Mn-C60 nanocomposites.
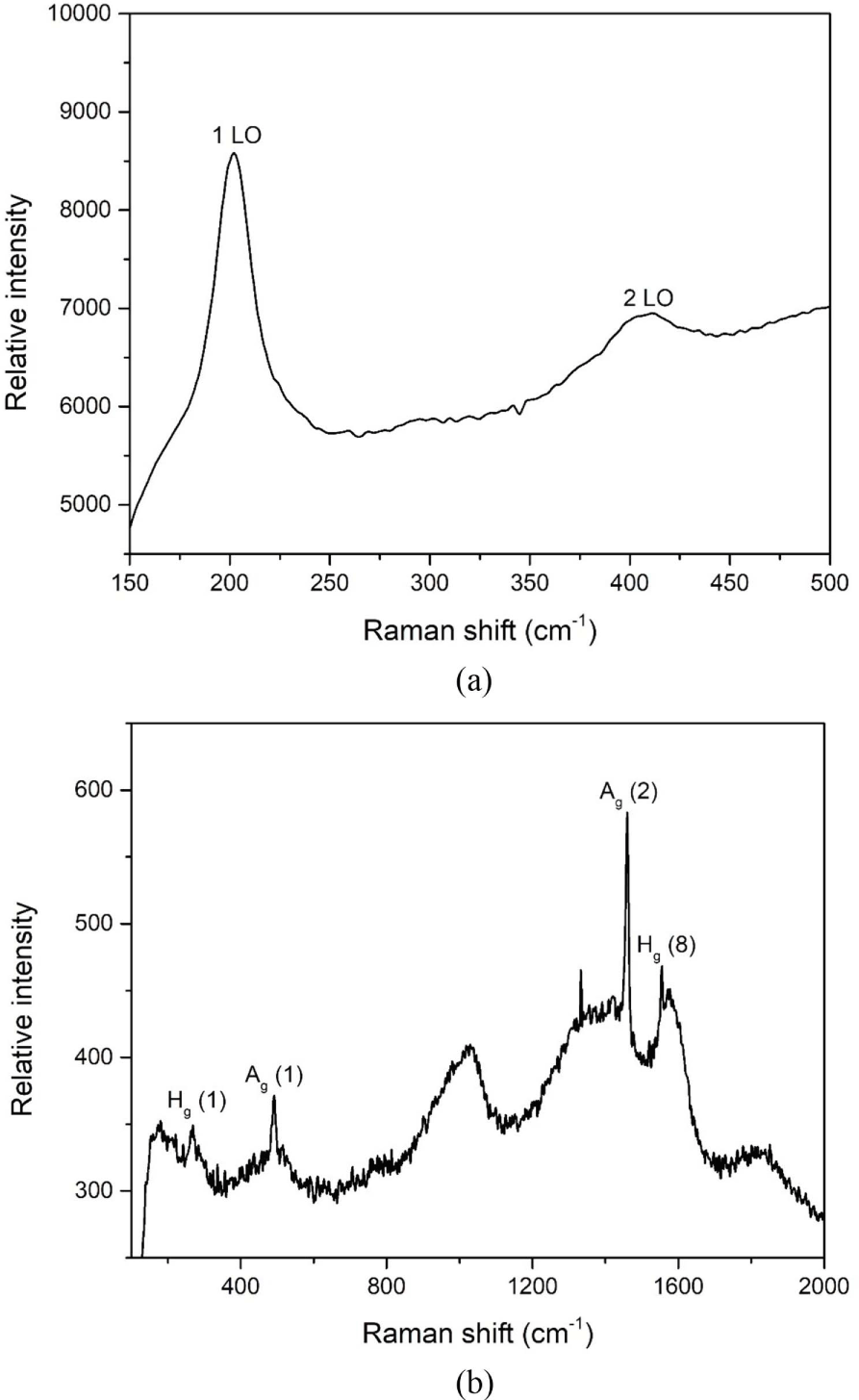
The photocatalytic performance of CdSe-Mn-C60 nanocomposites were estimated by the extent of organic dye degradation under 254 nm UV lamp irradiation. Figure 4(a-d) showed the UV-vis absorption spectra for decomposition of BG, MB, MO and RhB, respectively. CdSe-Mn-C60 nanocomposites effectively degraded all organic dyes except MO. In the photocatalytic degradation process, the main peaks occurred at 625 nm for BG, 665 nm for MB, 464 nm for MO, and 554 nm for RhB decreased with the increasing of irradiation time. The degradation percentages of organic dyes were calculated according to the Lambert-Beer law.11 After 80 min irradiation, 49.5% of BG, 77.5% of MB, 8.9% of MO, and 55.5% of RhB were degraded.
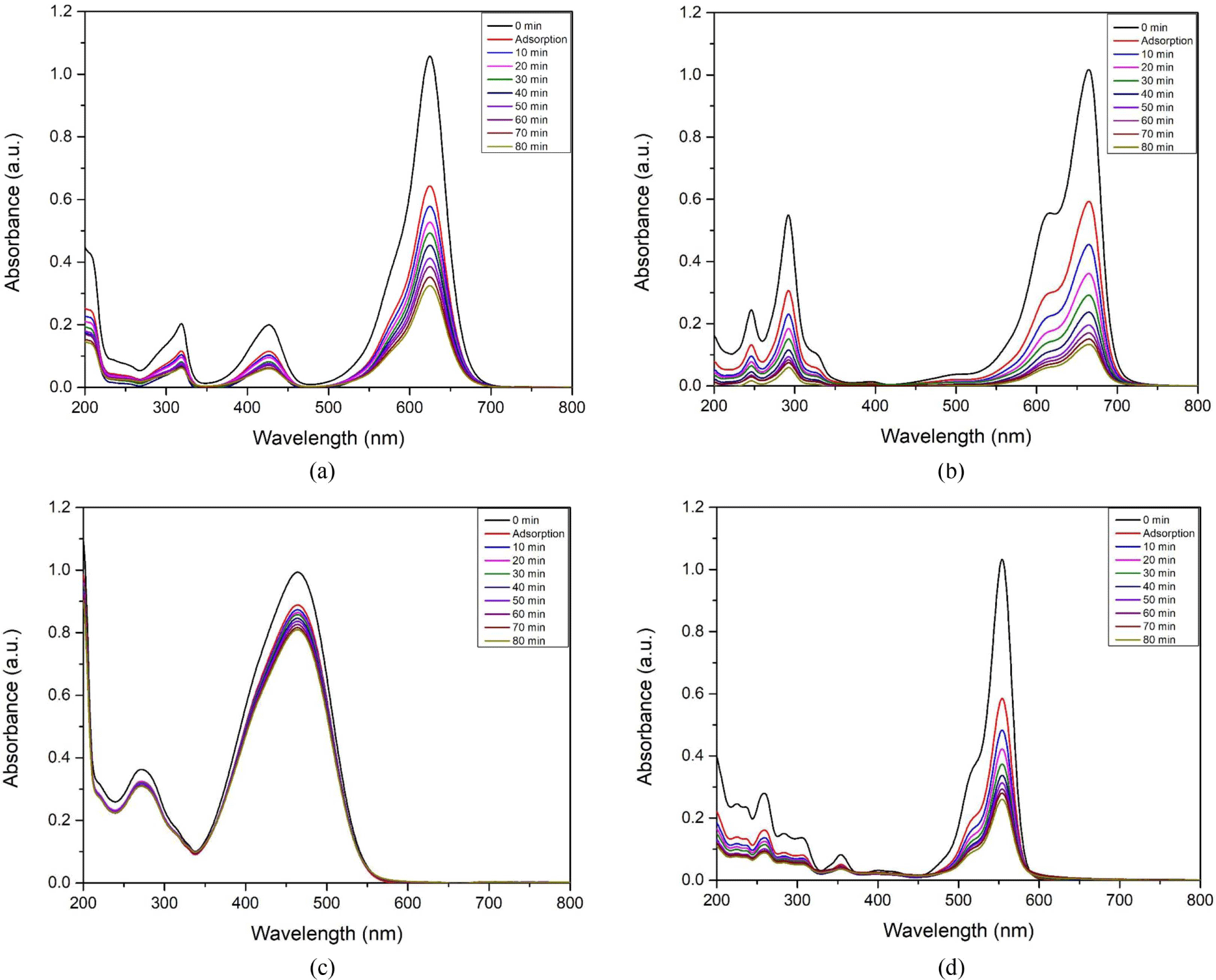
Langmuir-Hinshelwood rate model, as a model fit for pseudo-first-order kinetic processes have been used in previous works.1 To investigate the photocatalytic activity of CdSe-Mn-C60 nanocomposites, the kinetics of the photocatalytic decomposition were studied by employing Langmuir-Hinshelwood rate model. The equation is written as:
where C0 and C are the initial concentration of organic dyes solution and the concentration of organic dyes at time t. The rate constant is k, which can be calculated by the slop of lines. Figure 5 showed the variation of each organic dye concentration with time under 254 nm UV lamp irradiation by using 5 mg of CdSe-Mn-C60 nanocomposites as photocatalysis. The degradation rate of organic dyes using CdSe-Mn-C60 nanocomposites increased with the order of MO (0.0012 min-1) < BG (0.0084 min-1) < RhB (0.0096 min-1) < MB (0.0186 min-1).
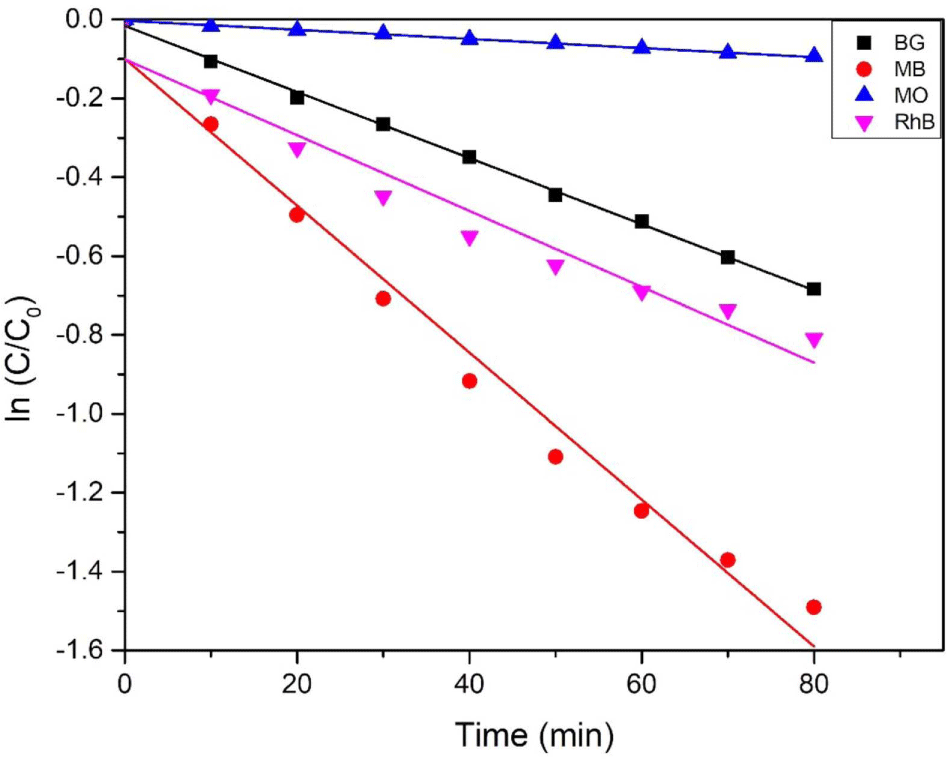
Conclusions
The CdSe-Mn-C60 nanocomposites were effectively prepared by a facile microwave irradiation method. The XRD pattern of the prepared nanocomposites suggested that the nanocomposites had a good crystalline structure. CdSe-Mn nanocomposites showed spherical and uniform morphology and fullerene (C60) displays flagstone-like structure with well distributed CdSe-Mn nanocomposites on the surface. The l LO, 2 LO vibration peaks of CdSe and Hg, Ag vibrational modes of fullerene (C60) were confirmed in Raman spectra, respectively. The photocatalytic efficiency of CdSe-Mn-C60 nanocomposites were estimated by measuring the extent of organic dye degradation under 254 nm UV lamp irradiation. The degradation rate of organic dyes increased with the order of MO (0.0012 min-1) < BG (0.0084 min-1) < RhB (0.0096 min-1) < MB (0.0186 min-1).