Introduction
The present harsh situation laid by the use of non-biodegradable plastics and their unconventional discarding practices is creating a thrust towards developing a green and sustainable environment.1-3 On the other hand, the common fillers used to develop plastic products are the carbonaceous materials derived from fossil fuels.4-7 Continuous depletion of fossils from the earth's crust becomes a great issue regarding the sufficient supply of these conventional filler materials. In this scenario, immense attention is being paid to alter the fossil-derived filler materials, especially with the waste materials to enable a circular economy and pollution-free environment. Eggshell (after the use of egg yolk and protein), being a bio-waste, degrades with time and pollutes the environment with a pungent smell.8-10 Use of the waste eggshell (containing outer calcite layer and inner collagen-based membrane) as filler material can be a sustainable and eco-friendly choice for making the “trash-to-treasure”, and a cost-effective approach towards promoting the circular economy while maintaining the biocompatibility and biodegradability of the developed products. Apart from these cost-effectiveness, eco-friendliness, biocompatibility, and biodegradability, the present trend of product development is to attract the customer's attention. In this regard, good tactile (or, touch-feeling) perception of the products is being considered as a potential factor to satisfy the customer's feelings and preferences towards purchasing the products.11-15 In this regard, nowadays, various biomaterials (especially waste biomaterials) are being attempted to incorporate in different products via various ways such as coating, finishing, texturing, filler incorporation, etc. to enhance their touch-feeling performance or comfort property. Realization of comfort property being a subjective assessment may vary from human to human because of the variation in human nature and the physicochemical properties of different human skins, which needs to simulate in an objective manner to realize the tactile property of different products in a more generalized and unbiased way.16-19 Various surface properties e.g., coefficient of friction (COF), average surface roughness (Ra), triboelectric property, etc. are being measured to realize the touch-feeling perception in a qualitative objective manner. In the case of triboelectric measurement, the effort has been emphasized to realize whether the developed material is tribo-positive or tribo-negative.14,19 From the triboelectric series, it has been observed that some of the biomaterials, e.g., fur, feather, human skin, hair, etc., showing tribo-positive characteristics are known to exhibit good touch-feeling performance.14,19-22 Considering the tribo-positivity and good touch-feeling properties of these biomaterials, attempts are being made to develop composites showing good tactile property using different bio-fillers. It has been observed that incorporating bio-fillers while enhancing the tribo-positive nature of the composites also reduces the COF. The reduction of COF is also considered to express improved tactile performance.14,19 Many attempts have been made to develop different products where the outer calcite part of ESM has been used as filler materials or coating agents.23-25 In the case of waste eggshell, other than the outer layer calcite part, there is an inner collagen-based membrane layer, which is also being recycled for different purposes ranging from cell culture template to battery separator.9,24,26-33 However, this collagen membrane (i.e., ESM), because of its complex crosslinked structure, is hard to process, particularly in the aqueous medium, which limits its applicability in broader areas of applications. In this regard, attempts have been made to isolate the eggshell protein from ESM.30,34,35 The isolated protein is found to be well dispersed in water and soluble in some of the common organic solvents (e.g., ethanol, acetic acid, etc.). Polyurethane (PU) is a common material in the coating industry; herein we have used waterborne polyurethane (WPU) to be well mixed with the soluble part of the eggshell membrane (S-SEM) to realize the formation of S-ESM/WPU bio-composite of enhanced tactile property and considerable mechanical property. Because of abundant proteinaceous functional groups of S-ESM, it is supposed that the composite may exhibit better tactile property similar to the other biomaterials (e.g., fur, feather, baby skin, etc.). Polar functionalities of the S-ESM filler are supposed to interact with the polar functionalities of the WPU matrix decreasing the availability of functional groups to interact with the frictionating objects, resulting in a decrease of the composite’s COF. Because of this polar-polar intermolecular interaction, it has been prejudiced that the rheology of the composite dispersion will be altered, and its mechanical property will remain nearly unaltered.
In this study, S-ESM/WPU composite films were prepared. The touch feeling performances in terms of COF and tribo-positive characteristics, along with the mechanical and thermal properties, were compared with those of the virgin WPU.
Experimental
The ester-type waterborne polyurethane (PUD 2008) having ~290 cps viscosity and milky white appearance was supplied by Aekyeong Chemical Co. Ltd. (S. Korea). The ESM after pulverization into 5 μm particle sizes using a cryogenic mill (IMP-400, Seishin Enterprise) under liquid nitrogen environment (−65°C to −70°C), was supplied by Sungkyun Biotech Co., LTD (S. Korea).
The overall process for extraction of S-ESM from ESM is schematically represented in Figure 1. The eggshell membrane is mainly composed of proteins containing various amino acids crosslinked via different peptide bonds. Probably because of this crosslinked network structure (mainly disulfide linked cysteine), the ESM remains non-dispersed in water.
The water-dispersible ESM was formulated via decrosslinking of its peptide bonds. To obtain this decrosslinked (or denatured) ESM, sodium hydroxide (NaOH) was used to break the peptide bonds of the ESM. The waste ESM powder (5 μm particle size) was dispersed in NaOH solution of water/ethanol (3:1) mixed solvent system and stirred for 3 hours at 60°C followed by stirring at room temperature for 24 hours till the ESM was completely dispersed. Then, the pH of the system was adjusted to 7.2~7.4 by dropwise adding dilute hydrochloric acid (HCl). After pH adjustment, centrifugation (at 9000 rpm) was performed to remove the undissolved ESM. Later, the inorganic impurities were removed via dialysis for 24 hours using a semipermeable membrane (Spectra/Por 3 dialysis membrane). The removal of Cl- was confirmed by adding the AgNO3 till the appearance of a white precipitate of AgCl disappeared. Following these multistep, the water-soluble ESM (S-ESM) was obtained. The solid form of this S-ESM was obtained just by drying the aqueous dispersion for 2 days at 55-60°C.
Later, the S-ESM powder at varying concentrations (i.e., 0.5, 1.0, 1.5 wt%) was simply added with WPU under mechanical agitation (i.e., stirring 1000 rpm) to prepare a homogenous dispersion of S-ESM/WPU. On the other hand, the ESM powders at any concentration were found to be aggregated, which limits the processing and preparation of ESM/WPU composite film.
The aqueous dispersions of S-ESM/WPU and virgin WPU were poured into the glass petri dish and dried at 40°C for 5 hours in a vacuum oven to obtain the films of WPU and S-ESM/WPU of 0.29 ± 0.05 mm thickness. For a comparative study, the preparation of ESM/WPU composite film of similar thickness was also accomplished. It is noteworthy to mention here that the drying temperature ≥ 50°C causes the unexpected formation of bubbles inside the film, which was found to be responsible for discrepancy or inhomogeneity during the characterization of these films.
The tactile property of the S-ESM/WPU composite was expected to improve with increasing the S-ESM content enriched with the proteinaceous materials. To explore this improvement, herein, we have investigated the in-plane COF and triboelectric property of the S-ESM/WPU bio-composite.
The in-plane COF was measured using the tactile meter (HEIDON Type 33, SHINTO Scientific) at room temperature of 22 ± 3°C, and 32 ± 5% relative humidity (RH). The tactile meter can adjust both the friction (under certain normal load in both the X and Y directions) and the sliding speed by controlling the jig with the help of the built-in software in the tactile meter. The tests were performed by sliding the specimens (20 mm × 20 mm) over the stage of the tactile meter by moving the jig to a sliding direction. All the tests were conducted under a normal load of 1N and a sliding speed of 10 mm/sec.
Conducting Cu-tape was attached with the bottom surface of virgin WPU and S-ESM/WPU films (20 mm × 20 mm) to make the device similar to the single electrode triboelectric nanogenerator (STENG) to be connected with the conducting port of the multimeter (2,450 Source Meter (Keithley, Tektronix), and fixed in the stage of the tactile meter. The film thickness was kept 0.29 ± 0.05 mm. The triboelectric measurement was performed at room temperature of 22 ± 3°C, and 32 ± 5% relative humidity by using tribo-negative poly tetrafluoroethylene (PTFE) sheet attached to the jig as an imparting object to find out the changes in the triboelectric property of the composite films in comparison to the WPU. The tribo-responses (i.e., output voltages) were obtained due to contact (with an impulse of 1 N) and separation of the frictionating surfaces controlled by the jig in an automatic fashion (to reduce the human experimental error).
The water contact angle of the S-ESM/WPU composite was investigated by using a contact angle analyzer (Phoenix 300 Touch, Surface Electro Optics Co., Ltd.) to observe the enrichment of polar functionalities over the composite surface with increasing the S-ESM content.
The morphological feature of the S-ESM/WPU composite was characterized by a scanning electron microscope (SEM) to discern the homogenous dispersion of S-ESM within the WPU matrix and compared with that of the ESM powder. To accomplish the formulation of homogenous composites, the precursor aqueous dispersion of S-ESM/WPU should be homogenous, which can only be obtained through abundant filler-matrix interaction. This interaction will lead to a change in the viscosity of WPU with varying the S-ESM content. To realize the filler-matrix interaction, herein, we have investigated the rheological property of aqueous dispersion of virgin WPU and S-ESM/WPU composites using a Brookfield viscometer DV2TRV (AMETEK Brookfield, USA). 180 ml of an aqueous dispersion of WPU and S-ESM/WPU was taken in a beaker, and the measurement was carried out at 25°C under 100 rpm using the spindle RV 02. The obtained viscosity was taken as the average of five experimental data.
The mechanical properties (i.e., the tensile strength and elongation at break) of the samples (0.29 ± 0.05 mm thick) were measured at a loading rate of 500 mm/min by a universal testing machine (Tensometer 2000, Myung Ji tech) following the standard ISO 37. An average value of five replicates was taken to conclude the mechanical property.
Differential scanning calorimetry (DSC) measurement was carried out using a DSC instrument (DSC Q20 (TA, American)) at a heating rate of 10°C/min within a range of 60°C to 200°C under nitrogen atmosphere. The glass transition temperature was recorded as the midpoint of the heat capacity change. Thermal stability was tested using a TGA (TGA Q50 (TA, American)). For this purpose, 10 mg of each test sample was heated from 25 to 600°C under a nitrogen atmosphere, with a heating rate of 10°C/min.
Results and Discussion
The ESM is a waste biomaterial mainly composed of collagen (containing disulfide-rich cysteine), glucosamine, and hyaluronic acid. NaOH, during extraction of S-ESM from ESM, breaks the sulfide bond, keeping other amino acids mostly unaffected. As a result, the cysteine content tends to decrease in S-ESM, resulting in the reduction of fishy odor (mainly arises due to the disulfide-rich cysteine) in S-ESM.
The extracted S-ESM, because of the de-crosslinking, is expected to possess more functional groups responsible for its well dispersion in the water, which, on the other hand, causes polar-polar interaction with the WPU functionalities (as schematically represented in Figure 2). Basically, during de-crosslinking, extracting, drying, and grinding, the ESM will be denatured, resulting in enhanced polar functionalities in S-ESM. The helical structure in ESM made of various interactions such as hydrogen (H)-bonding, covalent bonding, ionic interactions, disulfide bonding, non-polar interactions, etc., is supposed to be denatured during the processing of S-ESM, leaving various functional groups in the S-ESM.19
During mixing in the aqueous medium, the S-ESM functionalities interacted with the WPU functional groups mainly arises due to the hard segment, and occupied the interstitial spaces of WPU macromolecules, resulting in the formulation of homogenous precursor dispersion of S-ESM/WPU composite. The dispersion was found to be more flowable than that of the only WPU which may be due to the lowering of viscosity. Figure 3(a) demonstrates the feasibility of transparency and flexibility of the S-ESM/WPU composite film. From Figure 3(b), it can be observed that the viscosity of S-ESM/WPU aqueous dispersion was continuously reduced with increasing the S-ESM content. The viscosity was found to decrease from 290 mPa.s for virgin WPU to 117.6 mPa·s for 1.5 wt% S-ESM/WPU. The interaction among the molecules of WPU was reduced due to the inclusion of S-ESM (through the intermolecular H-bonding interaction) within the interstitial space among the WPU macromolecular chains, which is the reason behind lowering the viscosity of S-ESM/WPU dispersion.36 The lowering of viscosity (i.e., an increase of flowability) is supposed to be advantageous (i.e., time-resolved and ease in processibility) during coating of S-ESM/WPU composite dispersion over the surface of different objects. Due to the inclusion of S-ESM, it can be anticipated that the overall polar functionalities of the S-ESM/WPU composite will be enriched when compared with the virgin WPU, resulting in enhanced hydrophilicity (i.e., lowering of water contact angle) of the composite film. From Figure 3(c), it can be observed that the water contact angle decreases with increasing the S-ESM content. Representative pictures of contact angles of the composite with increasing S-ESM content are depicted in Figure 3(c). The decreasing trend of water contact angle with increasing the S-ESM content implies that the composite enriches with the polar functional groups with increasing the S-ESM content. As a consequence, well mixing of S-ESM with the WPU laid by the intermolecular H-bonding interaction, the S-ESM/WPU composites are expected to exhibit homogenous morphology under the microscope. From Figure 3(d), it can be observed that the composites exhibit homogenous morphologies for any amount of S-ESM content, whereas agglomeration was noticed for ESM at any content. Here, it is noteworthy to mention that the virgin ESM is not well dispersed in water, which causes improper and inefficient filler-matrix interaction. Because of the intermolecular interaction, the available polar functionalities of either WPU or S-ESM to interact with any other materials/objects decrease, resulting in the decrease of surface adhesion property of the composites.19 As a consequence, the average coefficient of friction (COF) felt by the S-ESM/WPU composite surface decreases with increasing the S-ESM (as shown in Figure 4). Compared to the virgin WPU and other composites, the composite containing 1.5 wt% of S-ESM shows the lowest value of COF. With increasing the S-ESM content, the composite film not only shows low COF value but also supposed to exhibit a better hydration effect (as evident by the contact angle measurement), which is a good indication of this composite film to be used as a coating layer over different materials showing attractive tactile property.
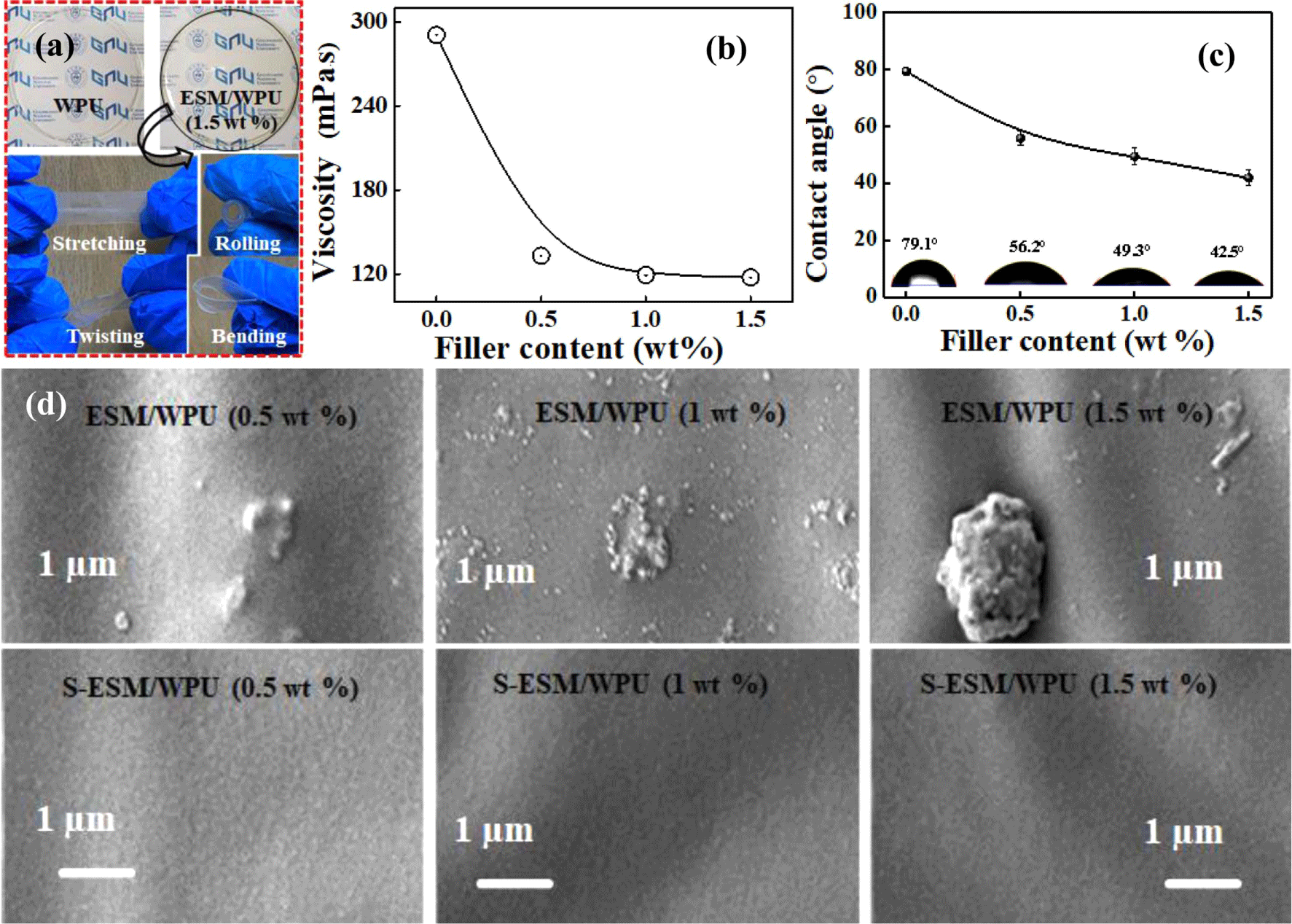
It has been observed that some biomaterials, e.g., fur, silk, feather, skin, hair, etc., exhibiting better touch-feeling sensation are tribo-positive in nature (as can be found in the triboelectric series).19-22 Considering this apparent relationship between the tactile property and triboelectricity, herein, we have approached the triboelectric investigation to realize the touch-feeling property of the composites. Because of the compositional similarity (especially the similar functional groups such as amine, amide, etc.) of S-ESM to that of the naturally abundant tribo-positive materials exhibiting better touch-feeling property, it is likely that the tactile property of the composite will be enhanced with increasing the S-ESM content. Reasonably, it is expected that the tribo-positive character of the composite will increase with increasing its S-ESM content.
The corresponding triboelectricity production mechanism has been schematically represented in Figure 5. When the PTFE and the STENG (made of S-ESM/WPU composite film attached over Cu-foil) are in contact, the overall charge is zero, and there is no production of voltage. However, as the most tribo-negative PTFE is separated, its contacting surface accumulates with negative charges (because of its electron-withdrawing fluoride groups), leaving positive charges on the surface of WPU and its composites. To equilibrate the induced positive charge, an opposite negative charge was developed on the Cu-electrode. This negative charge is a result of electrons flowing “from” the ground “to” the electrode. In this case, an upward peak (as depicted in Figure 5) has appeared as the tribo-response during separation. When the PTFE backs and contacts again with the STENG surface, some of the induced charges are neutralized, and the extra electrons “from” the electrode return back to the ground, resulting in a downward peak. As the response during contact is more material-dependent than that of the separation, where the abundant air can contribute more as interfering, in this present investigation, we are considering the response during contact only. With increasing the content of S-ESM, the tribo-positive character of the composite is expected to increase, resulting in enhanced output response during imparting of PTFE over the STENG surface.
Figure 6 shows the tribo-response (i.e., open circuit voltage) of the composite with increasing the S-ESM content when the composite surface was imparted with the tribo-negative PTFE. Figure 6 shows the representative graph exhibiting the response pattern of the STENG. From Figure 6, it can be seen that the response change of the virgin WPU is lower than those of the S-ESM/WPU 1.0 and 1.5 wt% which implies the increased tribo-positive character of the composite with increasing the S-ESM content. Thus, it can be said that the formation of a flexible tribo-positive PU composite having higher hydrophilicity laid by the well-mixing of S-ESM with WPU has been accomplished in this work. Considering, PTFE as most tribo-negative materials (because of most electronegative F-atom), the lower response among the virgin WPU and PTFE than that of the S-ESM/WPU and PTFE, infers that the WPU is tribo-negative materials while the composite shows increasing tribo-positive character with increasing the S-ESM content.
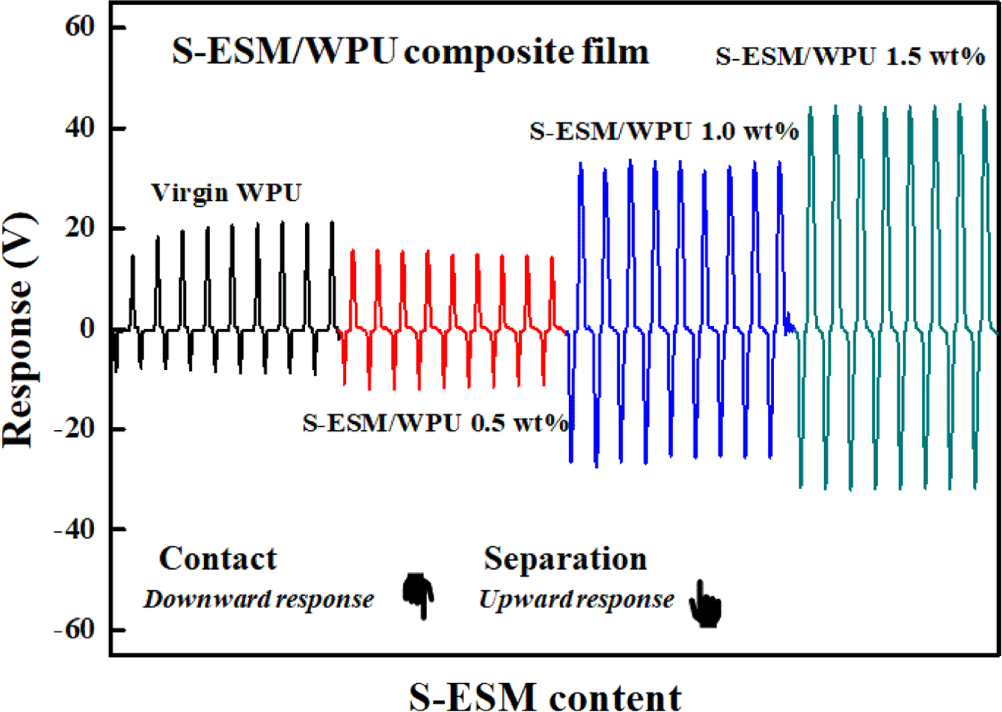
From the rheological observation, as it has been observed that the incorporation of S-ESM within the WPU matrix decreased the viscosity of S-ESM/WPU in contrast to the virgin WPU, it may be expected that the mechanical and thermal property of the composite will deteriorate with increasing the S-ESM content. Figure 7 shows the comparative mechanical (ultimate tensile strength and elongation at break) and thermal properties of the composite film. The results show that both the mechanical and thermal properties are not deteriorated. The proper dispersion because of sufficient intermolecular filler-matrix interaction may be the cause behind such observation.
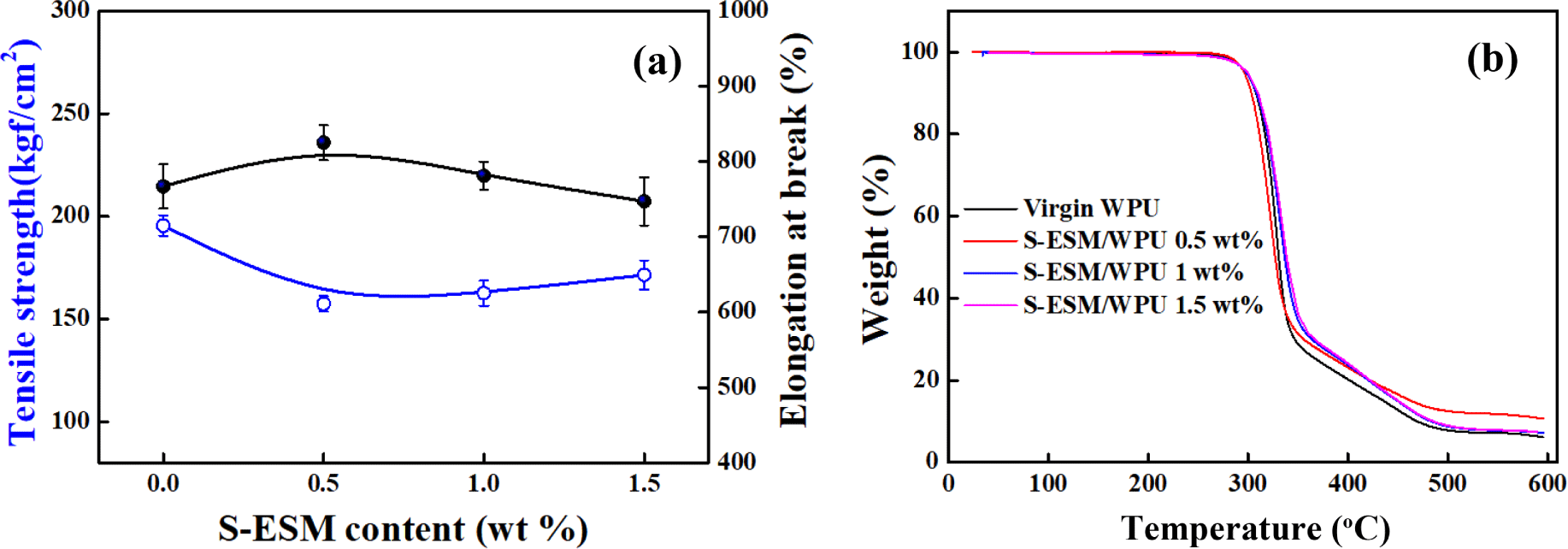
Conclusions
Recycling of waste ESM was accomplished via extraction of its soluble part (i.e., S-ESM) through sophisticated chemical and physical processes to develop a water-based dispersion with WPU. The S-ESM/WPU bio-composite film exhibits better tactile performance in terms of lowering the COF and raising the tribo-positive character with increasing the S-ESM content. The increased tribo-positive character can be considered as a cause of the reduction in the level of static electricity of the composite. Also, the incorporation of S-ESM was found not to deteriorate the thermal and mechanical properties of the composite when compared with the virgin WPU. Thus, it can be said that the composite can be considered for industrial upscaling as a future coating material (for automobile interiors and artificial leather) and artificial skin (for prosthetic and robotic applications).