Introduction
Tire wear particles (TWPs) are generated by friction between the tire tread and road surface, and the global production of TWP per year is estimated to be approximately 6 million tons.1-3 Various types of particles, like road pavement wear particles, road paint wear particles, and mineral particles, are found on roads. TWPs surrounded by these particles are referred to as tire-road wear particles (TRWPs). TRWPs flow into the environment and are detected in various environmental samples such as road dust, sediment, soil, and particulate matter.4-10
TWPs may contain potentially harmful chemicals that are released into the environment.11-13 Asphalt pavements contain bitumen that can release polycyclic aromatic hydrocarbons (PAHs) into the environment.14-16 PAHs are generated by incomplete combustion and can be adsorbed onto TRWP. Harmful heavy metals can also be adsorbed onto TRWPs. Road dust containing TRWPs is accumulated into rivers and seas and settle as sediment, and the sedimentation rate is proportional to its density and size.17-20 The sedimentation of TRWP affects aquatic systems, and harmful chemicals contained in TRWPs are steadily released into water. The sedimentation rate of a particle decreases with an increase in the surface area at the same density. TRWPs present on roads usually have densities > 1.2 g cm-3, and their densities depend on the degree of mineral particles adsorbed on the TWP surface.21,22 The TWP content in TRWPs is approximately 50 wt% on an average; however, it varies depending on the type and amount of other particles attached to the surface. The sedimentation rate of TRWPs in water is influenced by their density.
Ramp slopes in indoor parking garages are relatively steep, which leads to high friction and slip angle of tires while vehicles move. Ramps in indoor parking garages are typically made of cement concrete. Therefore, TRWPs generated in an indoor parking garage may have properties different from those produced on common asphalt-paved roads by moving vehicles. In this study, we collected TRWPs from road dust generated on 12 different sites on the ramp of an indoor parking garage (two ascent directions, two descent directions, and three different sites at down-, middle-, and up-locations) and characterized their physical properties, such as morphology and density. Each single TRWP was characterized. TRWPs generated from an asphalt-paved road (bus stop and taxi stand) were also sampled, and their properties were compared with those of the indoor parking garage.
Experimental
Road dust samples were collected at three different places, an indoor parking garage, a bus stop, and a taxi stand. The bus stop and taxi stand are all straight roads paved with asphalt, whereas the parking garage is paved with cement and vehicles are affected by slope and turning angles. The indoor parking garage is located in Sejong University, Republic of Korea (37°33’10.7”N 127°04’26.4”E), and the dust samples were collected on July 22, 2021. The bus stop is located on a six-lane road nearby Sejong University (37°32’59.1”N 127°04’32.3”E), and the dust sample was collected on July 14, 2021. The taxi stand is located about 1 km from Sejong University (37°33’7.4”N 127°05’21.8”E), and the dust sample was collected on July 26, 2021. Road dust samples were gathered by sweeping with a broom and stored in a glass vial.
For the indoor parking garage, dust samples were collected at twelve sites on the ascent and descent ramps (marked as A and D, respectively) as described in Figure 1. Each ramp was divided into six areas; the right and left sides for the vehicle’s moving direction (marked as R and L, respectively) and the down-, middle-, and up-locations in the ramp (marked as down, mid, and up, respectively).
The road dust samples were separated by size using a sieve shaker of Octagon 200 (Endecotts Co., UK). Standard sieves with aperture of 500, 212, 106, and 63 μm (Endecotts Co., UK) were used. Size separation was conducted with the interval mode for 20 min. There were rarely TRWPs in the road dust samples larger than 212 μm, and it is hard to select TRWPs from the road dust samples smaller than 106 μm. In the road dust samples of 106-212 μm, TRWPs were manually picked out through the help of microscope (EGVM 35B, EG Tech. Co., Republic of Korea). 30 TRWPs at least were selected for each sample. TRWPs were identified based on its elongated or wide appearance in black color, along with its elastic properties when pressed.
The density of a single TRWP was measued using NaBr and NaI aqueous solutions with the density ranging from 1.10 g cm-3 to 1.80 g cm-3 at interval of 0.1 g cm-3. NaBr and NaI were purchased from Ducksan Co. (Republic of Korea). The solutions of 1.10-1.50 g cm-3 were prepared using NaBr, and those of 1.60-1.80 g cm-3 were prepared using NaI. The density of a single TRWP was determined as follows (Figure 2): (1) pour 2 mL of the salt solution with a specific density into a 5 mL vial, (2) put a single TRWP into the vial, (3) gently shake the vial to sufficiently wet the TRWP with the solution, (4) leave for 30 min, (5) observe the state of TRWP in the solution whether float or sink, (6) transfer the TRWP to the salt solution of higher density when it is floated in the initial solution, (7) transfer the TRWP to the salt solution of lower density when it is sunk in the initial solution, (8) repeat the procedures (6) and (7), and (9) determine the density of a single TRWP.
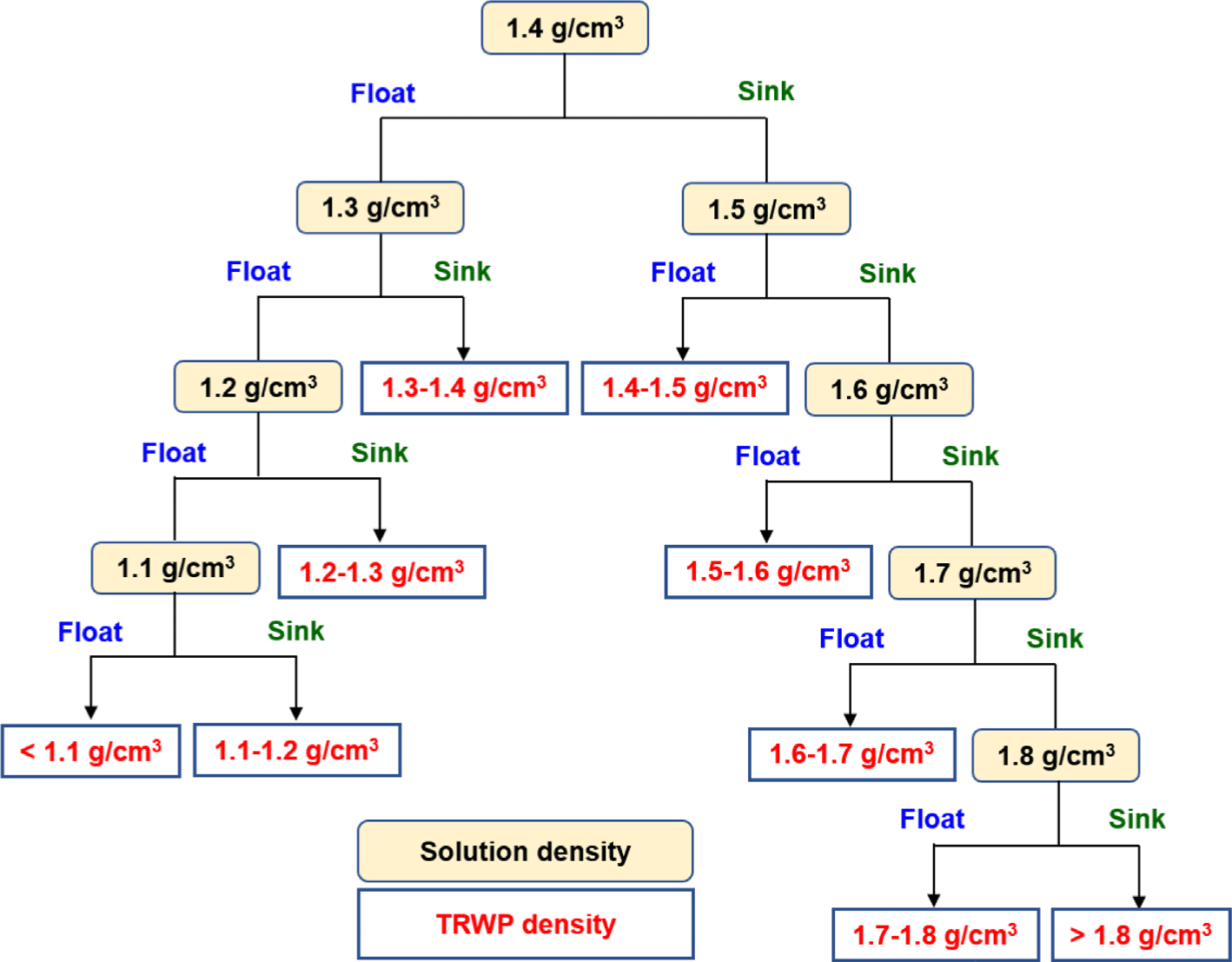
The morphology of TRWP was observed using a digital microscope (DM4M, Leica Microsystems, Germany). The Z stack and tilescan functions of the LAS X program provided by Leica were used. The lengths of the major and minor axes of TRWP were measured using the 2D analysis module, and the aspect ratio was calculated.
Results and Dscussion
The TRWPs were categorized by their densities ranging from < 1.1 to > 1.8 g cm-3, and divided into nine groups with the interval of 0.1 g cm-3, and their magnified images are shown in Figure 3. The TRWPs collected from the indoor parking garage contained particles of all nine groups; however any sampling site with TRWPs of all groups was not observed. In the PDR sample, the TRWPs > 1.8 g cm-3 were detected, but those < 1.1 g cm-3 were not observed. TRWPs < 1.1 g cm-3 were detected in samples collected from other sampling sites in the indoor parking garage. For the PAR and PDL samples, TRWPs > 1.7 g cm-3 were not detected. The TRWPs collected from the asphalt-paved road had a relatively narrow density range between 1.2-1.8 g cm-3. TRWPs collected from the bus stop (BS) had a relatively higher density (1.3-1.8 g cm-3) than those collected from the taxi stand (TS) (1.2-1.6 g·cm-3).
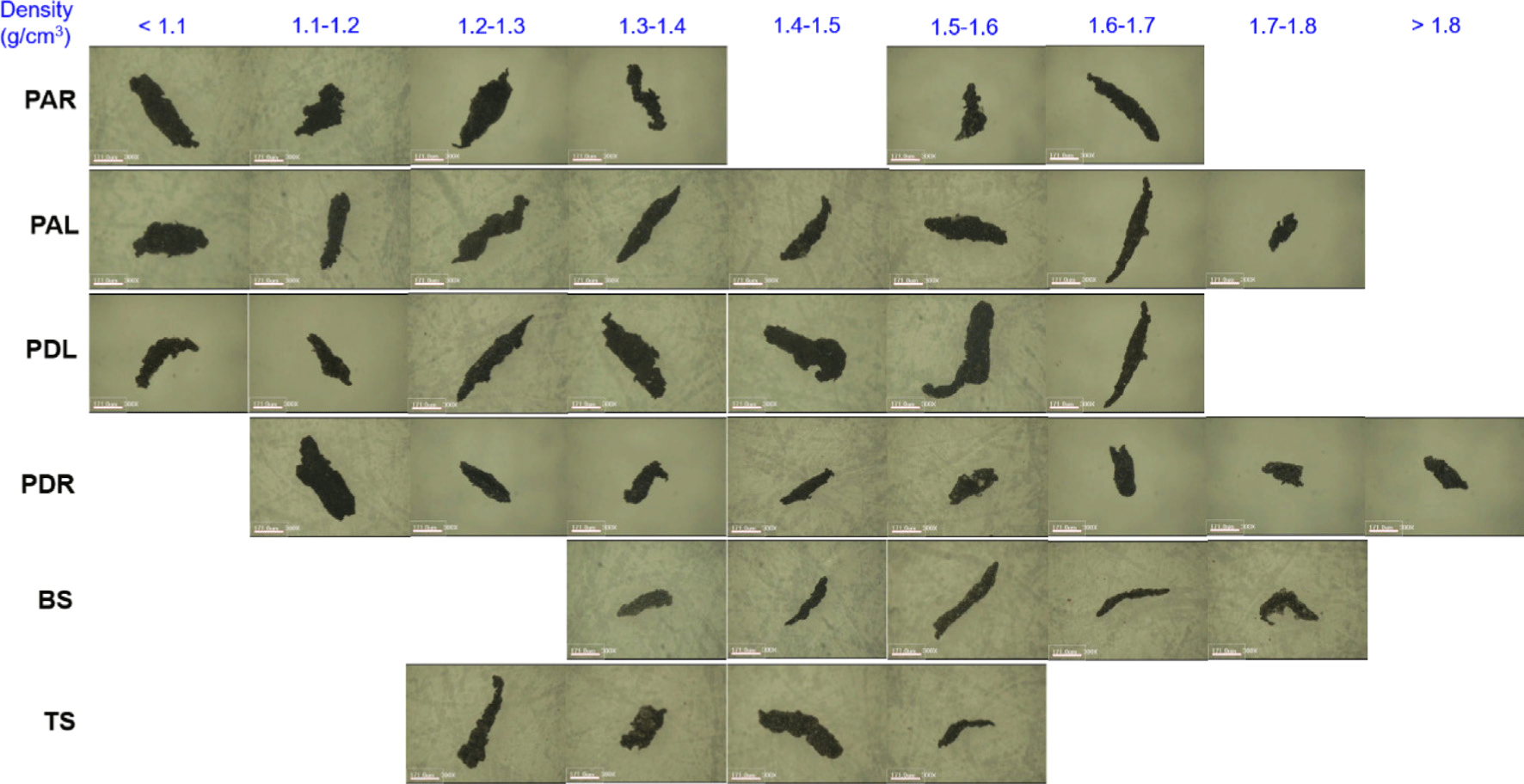
A lot of mineral particles were observed on the surface of TRWP (Figure 4). The sizes of the mineral particles varied from submicrometers to several tens of micrometers. Most mineral particles were smaller than 10 μm. The number of mineral particles on the TRWP surface increased as the density of TRWP increased. Therefore, the density of TRWP is determined by the number of mineral particles.
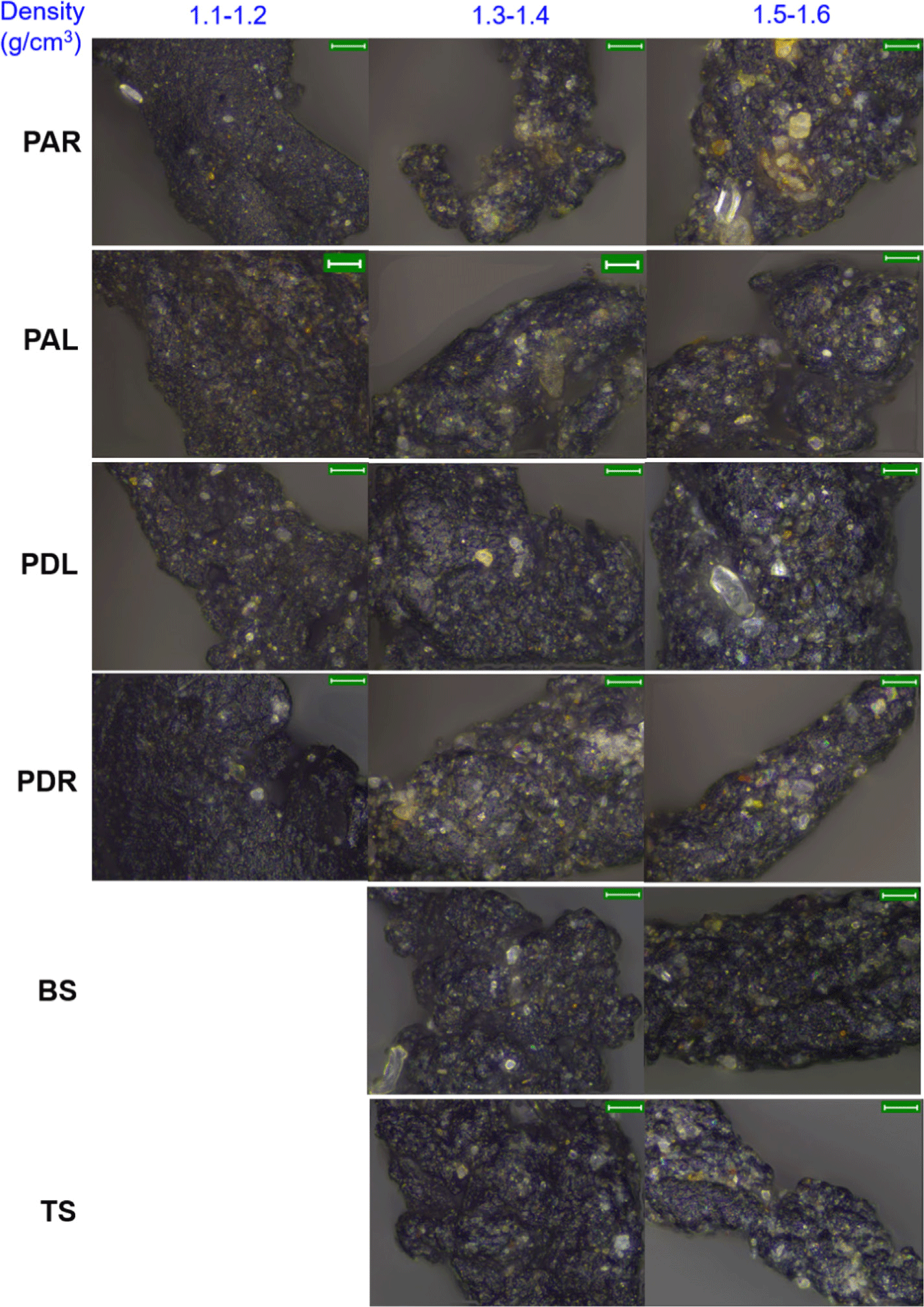
Variations in the density distributions of TRWPs collected from the indoor parking garage are shown in Figure 5. Density distribution of the PAR and PAL samples showed different patterns; the PAR sample distribution peaked at the lowest density (< 1.1 g cm-3) and then decreased with an increase in the density, whereas the PAL sample distribution peaked at the density of 1.2-1.3 g cm-3. More than 50% of the PAR sample was distributed at the lowest density (< 1.1 g cm-3), and all TRWPs were distributed at the density < 1.3 g cm-3, except for the PAR-down sample. The TRWPs with a density < 1.3 g cm-3 indicate that they contain mineral particles in a small amount because TRWPs are generated by short-term contact between the tire tread and road surface. Density distribution of the PAR-up sample dramatically decreased from 70% to 7% when the density increased from < 1.1 g cm-3 to 1.2-1.3 g cm-3, while that of the PAR-mid sample notably decreased from 63% to 7%. Most TRWPs in the PAR-down sample were distributed in a low-density range of < 1.3 g cm-3; however 10% TRWPs were distributed in a relatively high-density range of 1.3-1.7 g cm-3. Some TRWPs present on the surface of tire tread may be released by friction when a vehicle enters an ascent ramp, and some cracks on the tire tread can be detached by high friction. These TRWPs would have a relatively high density > 1.3 g cm-3.
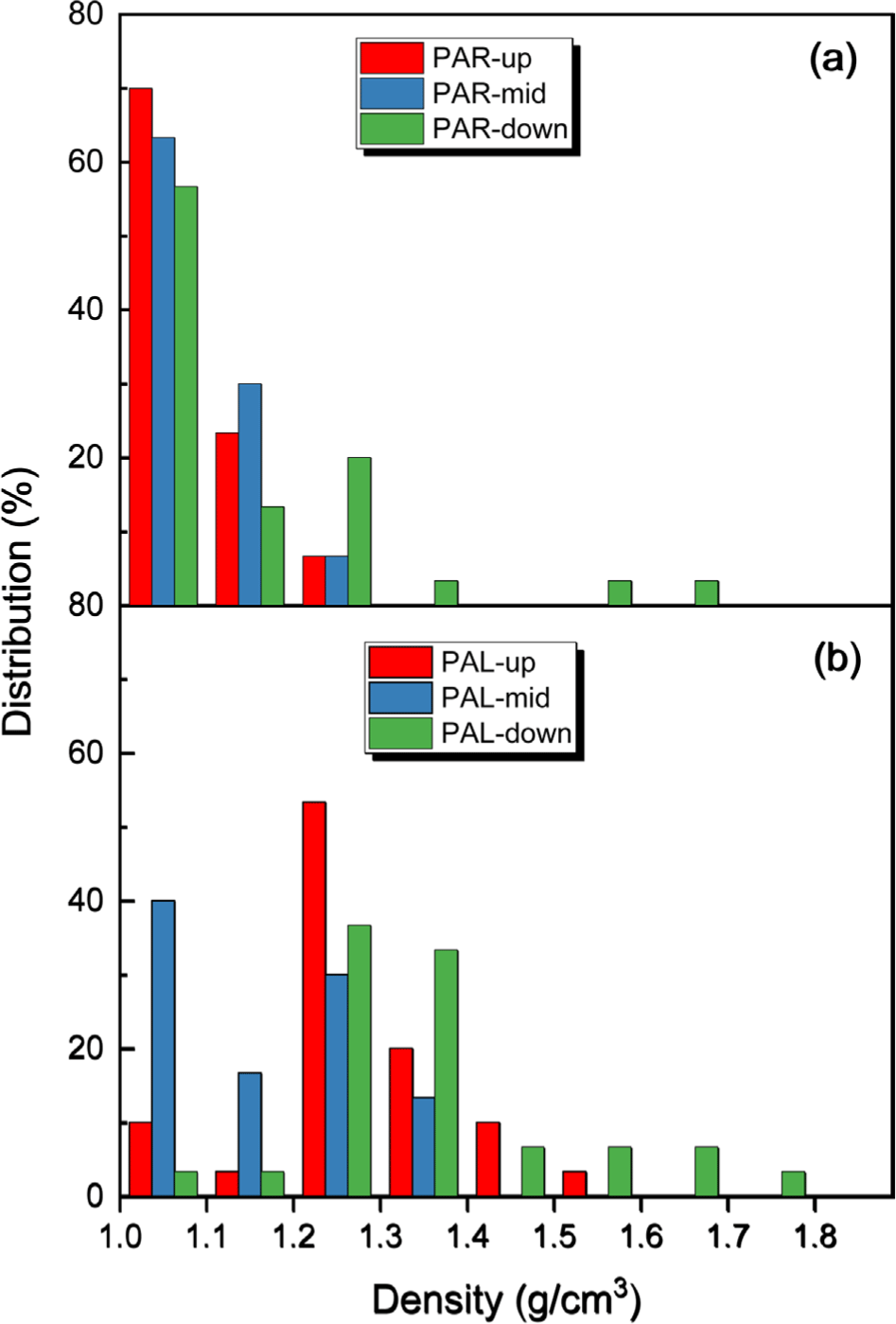
Density distribution of the PAR sample shifted to a lower density than that of the PAL sample, which may have been owing to the difference in slip angles between tires located on the right and left sides. The slip angle of the tire on the right side (PAR) of an ascending vehicle is greater than that of the tire on the left side (PAL), and a high slip angle can lead to more abrasion of the tire tread within a short time.
Short-term contacts can reduce the contact time with mineral particles on the ascent ramp and the number of mineral particles attached to the TRWP surface. Density distribution of TRWPs in the PAL-up sample peaked at 1.2-1.3 g cm-3 and then decreased as the density increased. The densities of the PAL-mid sample were distributed at a low and narrow density range of < 1.4 g cm-3 without specific trend of the distribution, and 30% and 40% particles were distributed at the densities > 1.1 g cm-3 and 1.2-1.3 g cm-3, respectively. The densities of the PAL-down sample were distributed at a wide range from < 1.1 g cm-3 to 1.7-1.8 g cm-3, and 70% being at the 1.2-1.4 g cm-3.
Variations in the density distribution of TRWPs collected at the descent ramp are shown in Figure 6. Density distributions of the PDR and PDL samples showed different patterns; the PDR sample peaked at the density range of 1.2-1.4 g cm-3, whereas the PDL sample peaked at the lowest density (< 1.1 g cm-3). Approximately 77%, 80%, and 65% TRWPs in the PDR-up, PDR-mid, PDR-down samples, respectively, were distributed at the density of 1.2-1.3 g cm-3. The TRWPs in the PDR-up and PDR-mid samples were distributed in a low density range of 1.1-1.6 g cm-3, whereas those in PDR-down sample were distributed in a high density range > 1.2 g cm-3. One TRWP with the highest density > 1.8 g cm-3 was observed.
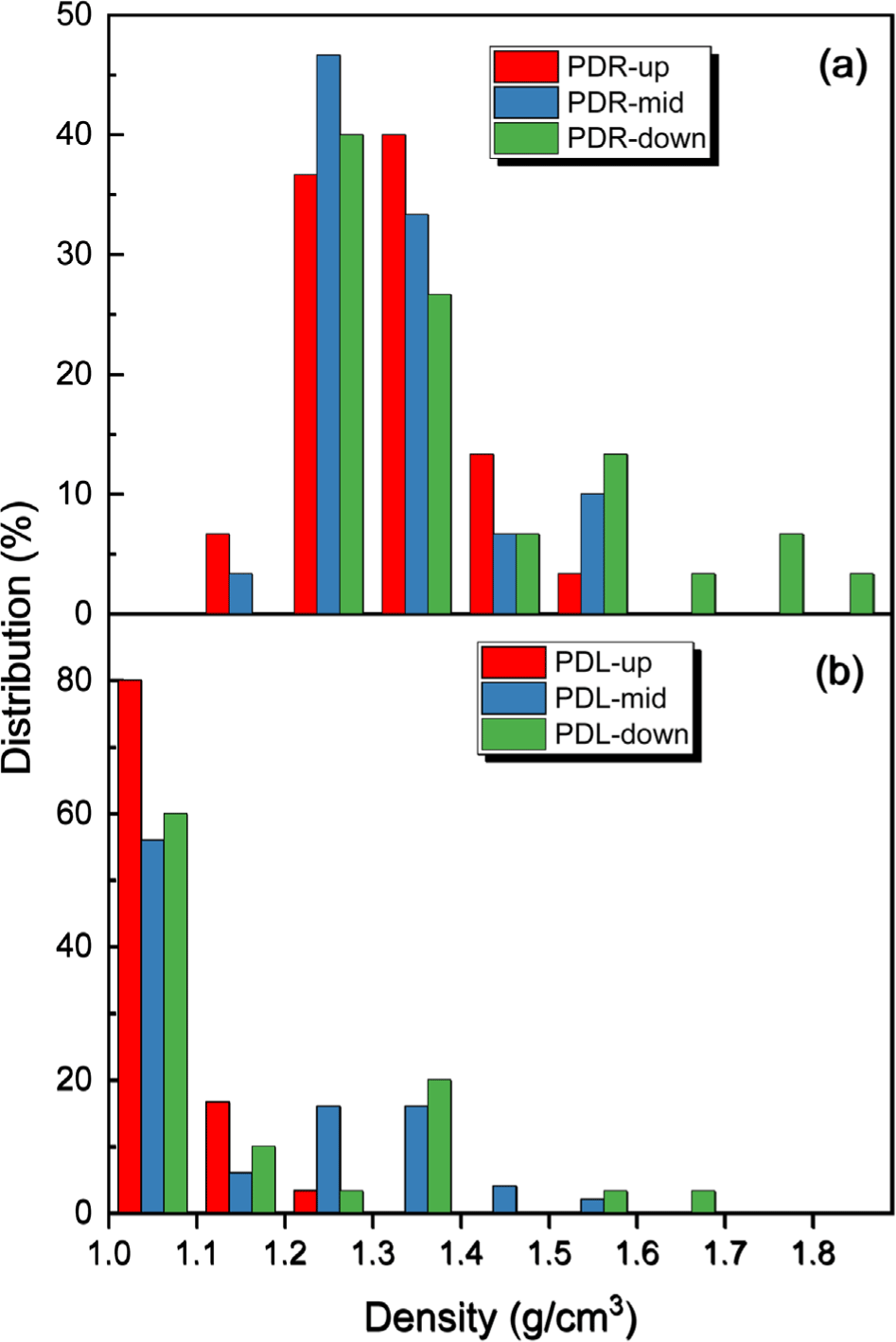
Approximately 80%, 56%, and 60% TRWPs in the PDL-up, PDL-mid, and PDL-down samples, respectively, were distributed at the lowest density < 1.1 g cm-3. The TRWPs in the PDL-up sample were distributed in a low and narrow density range < 1.3 g cm-3, whereas those in the PDR-mid sample were distributed in a low but relatively wide density range < 1.6 g cm-3. The TRWPs in the PDL-down sample were distributed in a relatively high density range < 1.7 g cm-3; however, the TRWPs with density of 1.4-1.5 g cm-3 were not detected. The TRWPs with the high density > 1.7 g cm-3 were not observed in the PDL samples. The density distribution of TRWPs in the PDL sample was similar to that in the PAR sample, whereas that in the PDR sample was similar to that in the PAL sample.
The TRWPs with low density < 1.2 g cm-3 were not observed in the BS and TS samples (Figure 7). The density range of TRWPs in the TS sample (1.2-1.6 g cm-3) was lower than that in the BS sample (1.3-1.8 g cm-3). The distribution of TRWPs in the BS sample increasd from 23% to 36% as the density increased from 1.3-1.4 to 1.5-1.6 g cm-3, and then decreased to 3% at 1.7-1.8 g cm-3 density. The distribution of TRWPs in the TS sample increasd from 17% to 37% as the density increased from 1.2-1.3 to 1.5-1.6 g cm-3. The density distributions of TRWPs in the BS and TS samples shifted to a higher density range than those in the parking garage samples (PAR, PAL, PDR, and PDL samples) because TRWPs in the BS and TS were generated through relatively long contact times between the tire tread and road surface compared to the contact times for generating TRWPs in the parking garage.
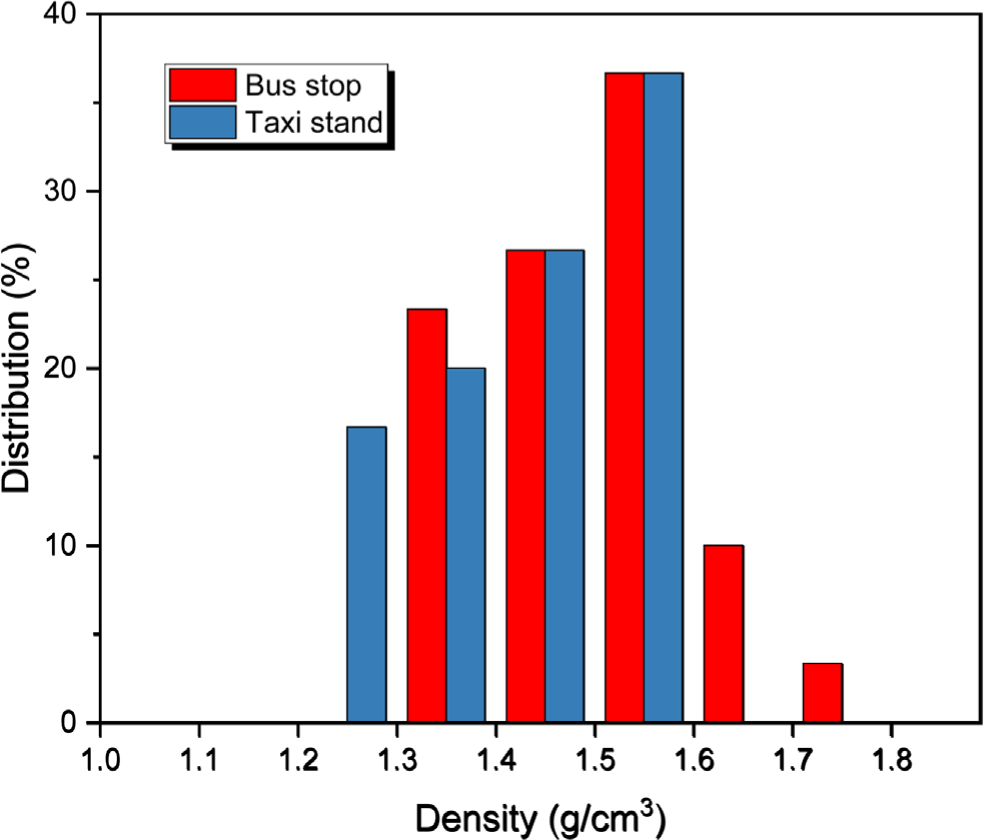
The aspect ratios of TRWPs affect their surface area and sedimentation rate in water. The aspect ratios of TRWPs collected from the 12 sampling sites in the indoor parking garage were plotted as a function of density (Figure 8). The TRWPs showed a wide range of aspect ratios, which varied depending on their densities and sampling sites. The ranges of aspect ratios for the ascent and descent ramps were similar; however, the ranges with most TRWPs for the descent ramp (2.0-6.0) were wider than those for the ascent ramp (2.0-5.0).
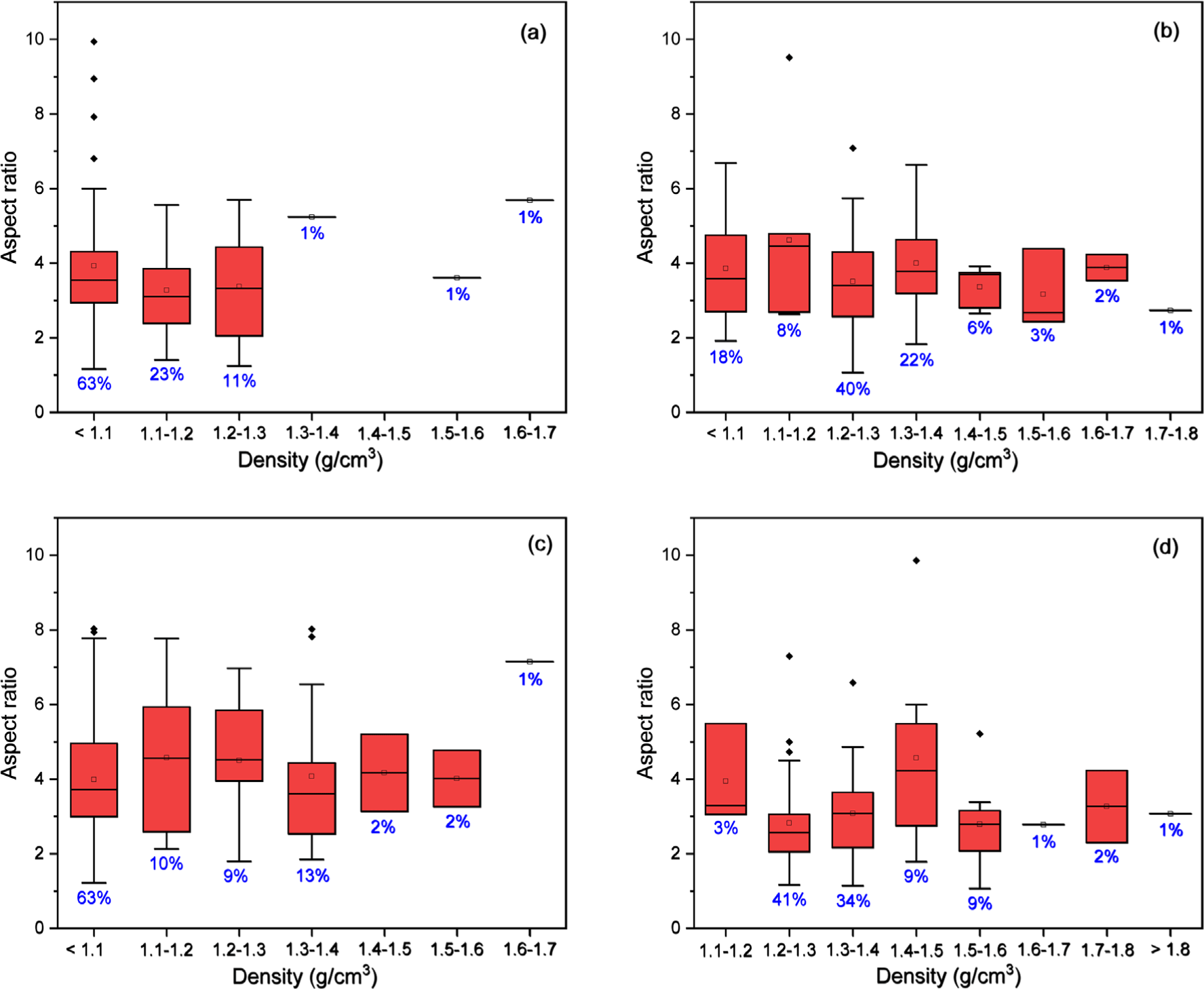
For the ascent ramp, the tires located on the right side of the vehicle (AR) had slip angles higher than those located on the left side (AL). The ranges of aspect ratios for the AR samples (1.2-9.9) were slightly wider than those for the AL samples (1.1-9.5), and ranges with most TRWPs for the AR samples (2.0-5.0) were also slightly wider than those for the AL samples (2.5-5.0). For the AR samples, the 63% TRWPs had the lowest density < 1.1 g cm-3, and the range of aspect ratios changed from 1.2-9.9 through 1.4-5.6 to 1.2-5.7 as the density increased from < 1.1 g cm-3 to 1.2-1.3 g cm-3. For the AL samples, the most distributed TRWPs (40%) had the density of 1.2-1.3 g cm-3, and the range of aspect ratios was 1.1-7.1.
For the descent ramp, the tires located on the left side of the vehicle (DL) had slip angles higher than those located on the right side (DR). The ranges of aspect ratios for the DL samples (1.2-8.0) were narrower than those for the DR samples (1.1-9.9), and the ranges with most TRWPs for the DL samples (2.5-6.0) were also slightly narrower than those for the DR samples (2.0-6.0). For the DL samples, low-density (< 1.3 g cm-3) TRWPs had a wider range of aspect ratios than those of the other TRWPs. The range of aspect ratios became narrow as the TRWP density increased. The most distributed TRWPs (63%) had the lowest density (< 1.1 g cm-3) and a wide range of aspect ratio (1.2-8.0). For the DR samples, no specific trend was observed in changes of the aspect ratios of TRWPs with respect to density. The distribution of TRWPs with density ranges 1.2-1.3 and 1.3-1.4 g cm-3 were 41% and 34%, respectively, and the ranges of their aspect ratios were 1.2-7.3 and 1.1-6.6, respectively. Variations in the aspect ratios of TRWPs were examined for the down-, mid-, and up-position sampling sites (Figures 9, 10, and 11, respectively: Supplementary material). For the down-position (Figure 9), the range of aspect ratios for the samples of descent ramp (1.6-9.9) was wider than that for the samples of ascent ramp (1.2-6.0). The range of aspect ratios for the AR-down sample (1.2-6.0) was wider than that for the AL-down sample (1.5-5.7), and the range with most TRWPs for the AR-down sample (2.0-4.0) was narrower than that for the AL-down sample (1.8-4.3). The range of aspect ratios for the DL-down sample (1.8-8.0) was narrower than that for the DR-down sample (1.6-9.9), and the range with most TRWPs for the DL-down sample (2.2-5.4) was wider than that for the DR-down sample (2.2-4.3).
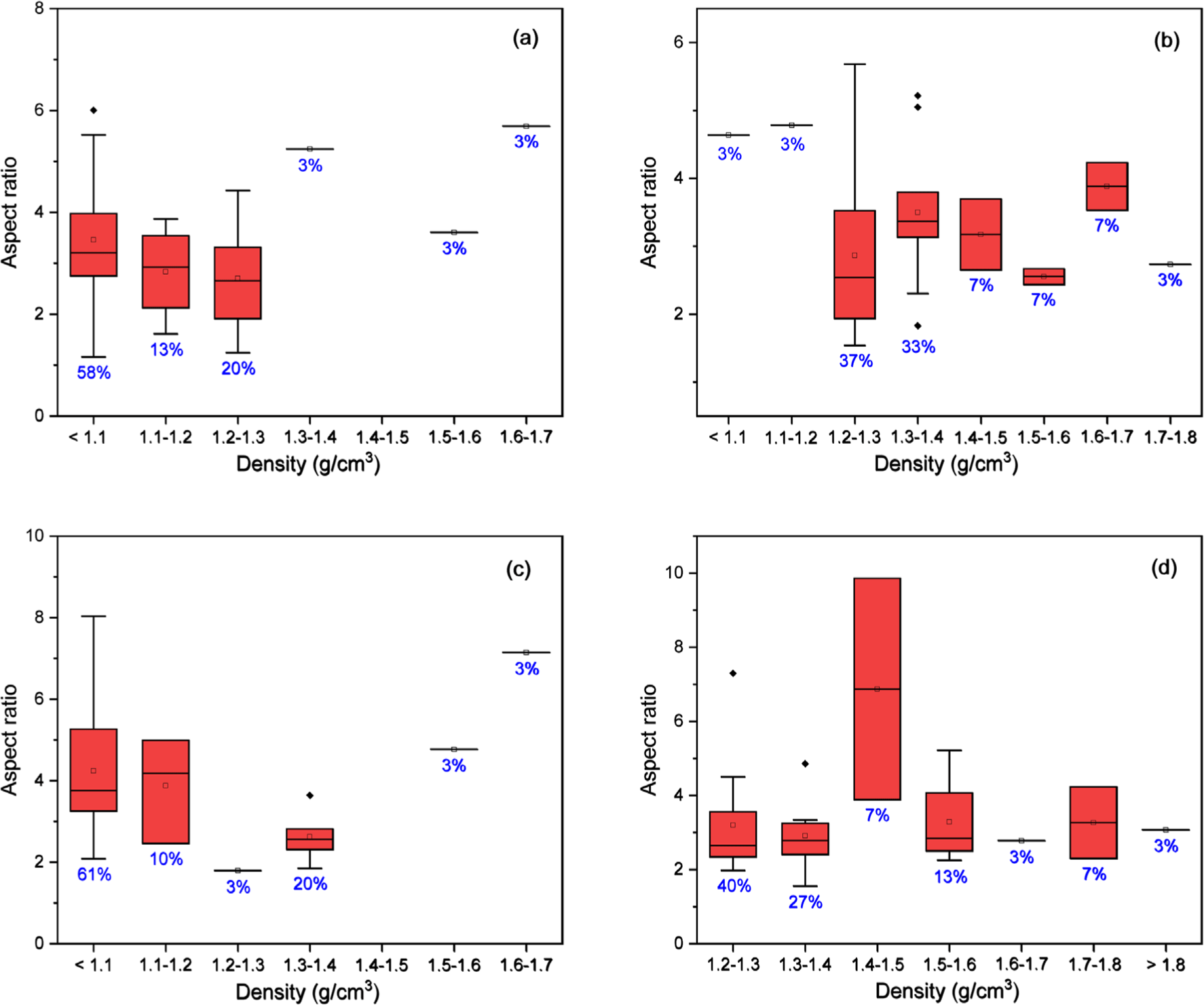
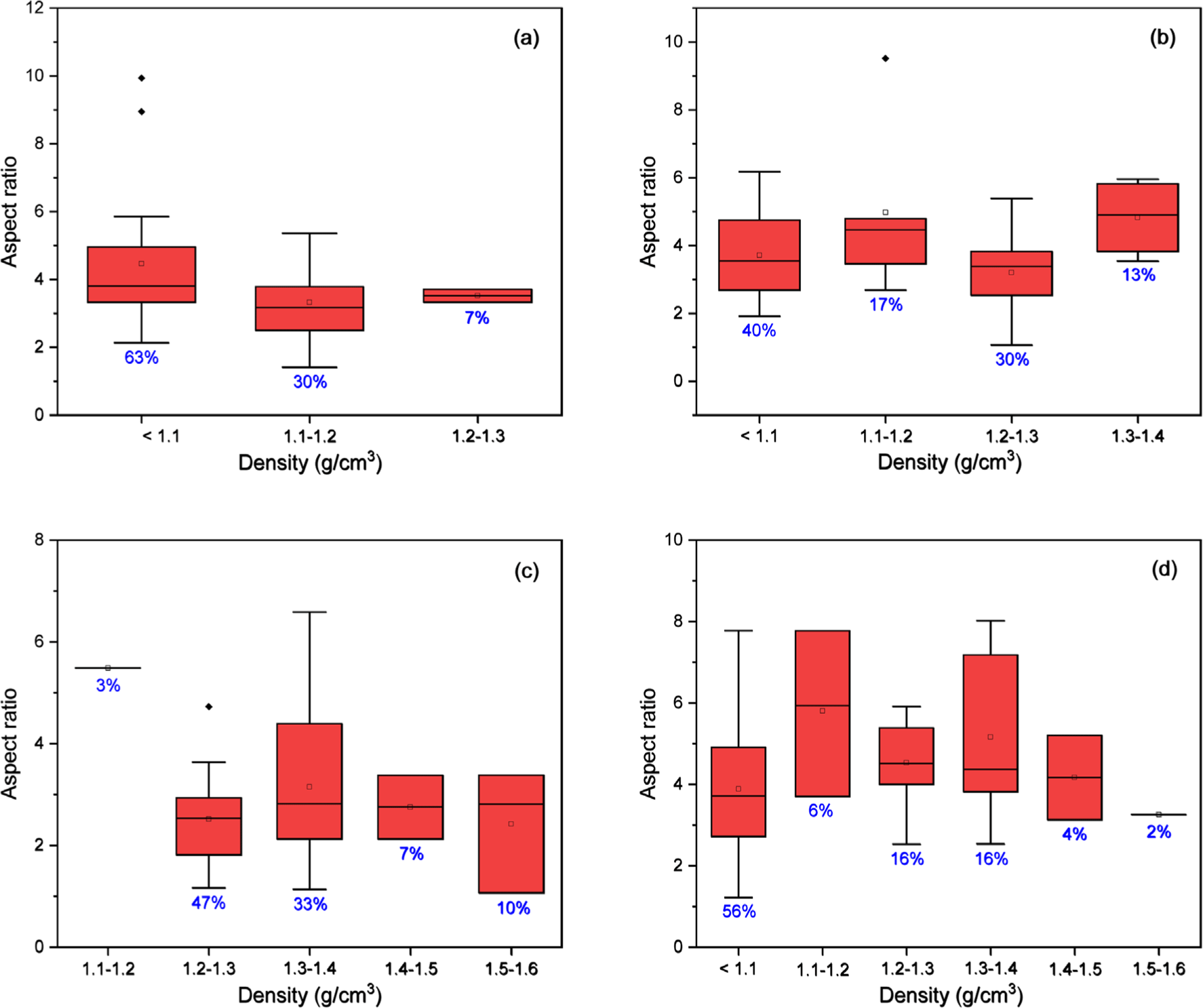
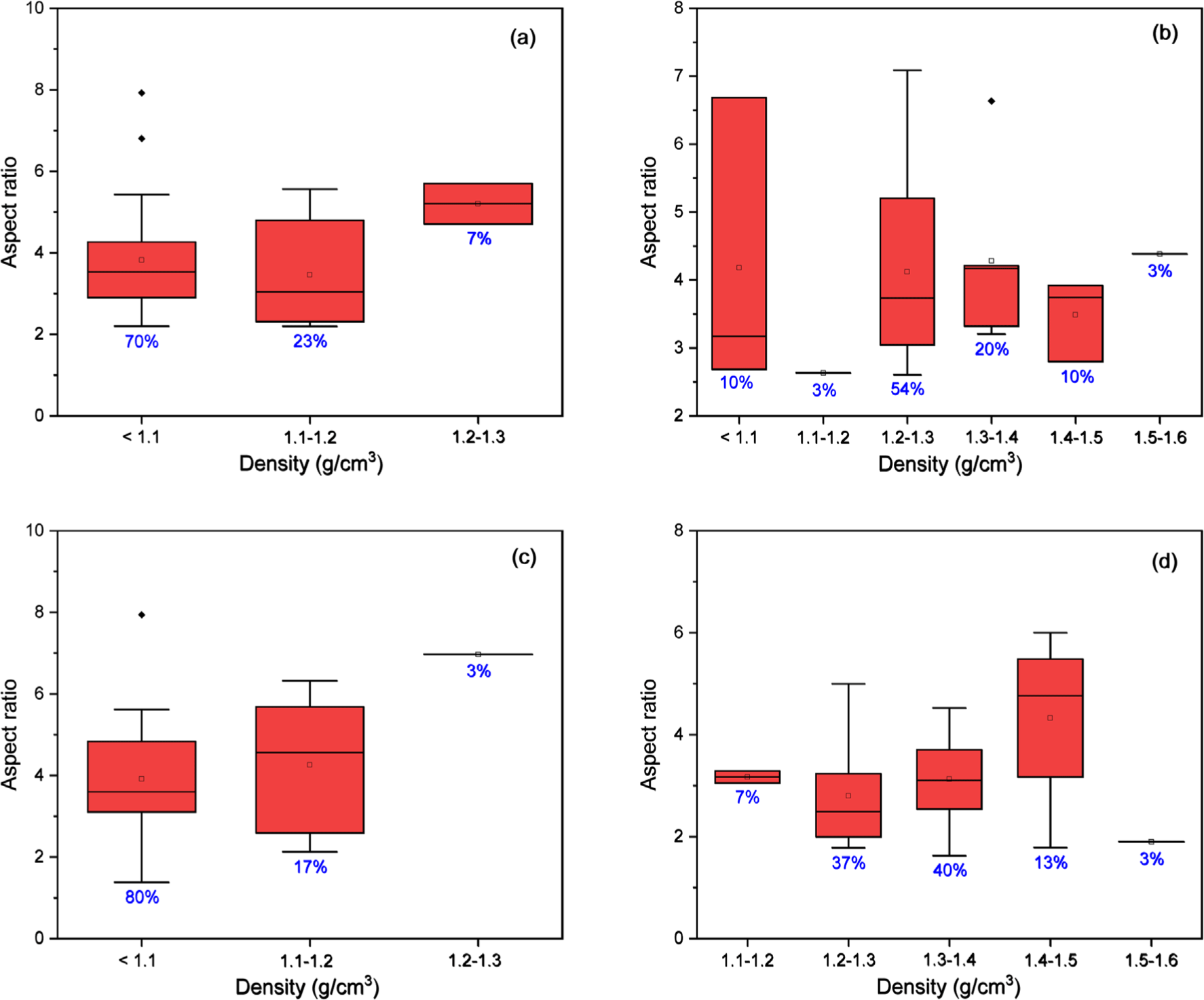
For the mid-position (Figures 10), the ranges of aspect ratios for the ascent-ramp samples (1.1-9.9) were wider than those for the descent-ramp samples (1.1-8.0). The ranges of aspect ratios for the AR-mid (1.4-9.9) and AL-mid (1.1-9.5) samples were similar. The range of aspect ratios for the DL-mid sample (1.1-6.6) was narrower than that for the DR-mid sample (1.2-8.0), and the range with most TRWPs for the DL-mid sample (1.1-4.0) was also narrower than that for the DR-mid sample (2.7-7.2). For the up position (Figure 11), the ranges of aspect ratios for the descent-ramp samples (1.4-7.9) were wider than those for the ascent-ramp samples (2.2-7.9).
The range of aspect ratios for the AR-up sample (2.2-7.9) was wider than that for the AL-up sample (2.6-7.1), and the range with most TRWPs for the AR-up sample (2.3-5.0) was narrower than that for the AL-up sample (2.6-6.7). The range of aspect ratios for the DL-up sample (1.4-7.9) was wider than that for the DR-up sample (1.6-6.0).
Variations in the aspect ratios of TRWPs collected at the BS and TS are shown in Figure 12. The aspect ratios of TRWPs collected at the BS and TS (2.9-11.2 and 2.4-13.4, respectively) were higher than those of TRWPs collected in the indoor parking garage (1.1-9.9) probably because of more secondary friction in the BS and TS than in the indoor parking garages. For the TRWPs in the BS sample, the aspact ratio and its range increased as the density of TRWPs increased; the range of aspect ratios changed from 3.0-6.3 to 6.8-10.7 as the density increased from 1.3-1.4 g cm-3 to 1.6-1.7 g cm-3. For the TRWPs in the TS sample, the aspact ratio and its range increased as the density of TRWP increased, except for the TRWPs of 1.2-1.3 g cm-3; the range of aspect ratios changed from 2.4-7.3 to 2.5-10.5 as the density increased from 1.3-1.4 g cm-3 to 1.5-1.6 g cm-3.
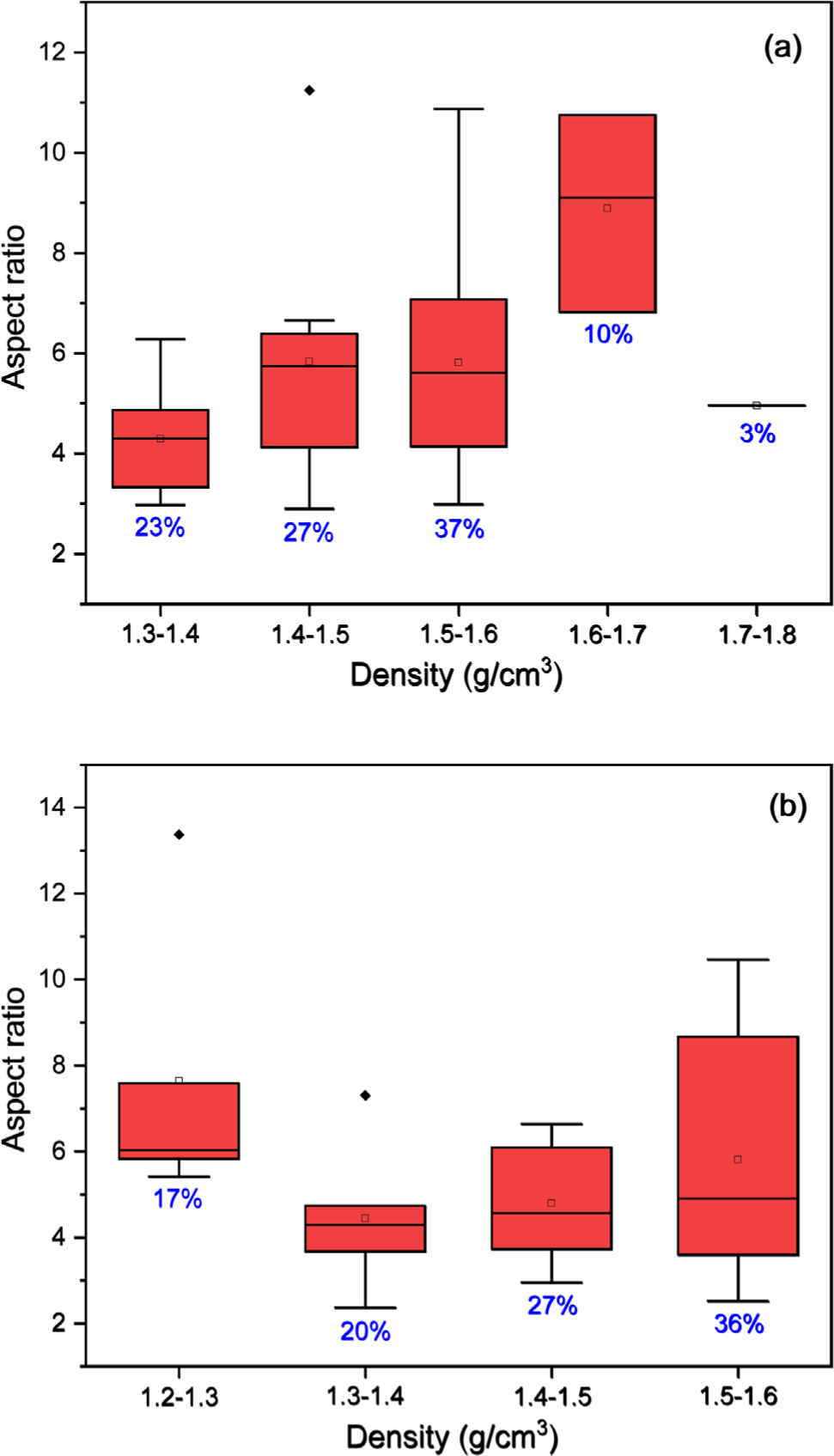
Conclusions
The findings of this study clearly indicated that TRWPs produced on the indoor parking garage ramps and asphalt-paved roads had different characteristics owing to the differences in contact times to form TRWPs and slip angles of tires. The contact time is shorter and the slip angle is larger in the indoor parking garage ramp than in the BS and TS. The TRWPs in the indoor parking garage had wide density ranges from < 1.1 g cm-3 to > 1.8 g cm-3, while those in the BS and TS samples had relatively narrow density range of 1.2-1.8 g cm-3. More than 50% TRWPs produced on the parking garage ramp had the lowest density (< 1.1 g cm-3). The number of mineral particles attached on the TRWP surface increased as the density of TRWP increased. The aspect ratios of TRWPs collected at the BS and TS were higher than those of TRWPs collected in the indoor parking garage probably because of more secondary friction in the BS and TS than in the parking garage. The densities and aspect ratios of TRWPs generated in the parking garage varied depending on the ascent and descent ramps and slip angles of tires. The aspect ratios of TRWPs affect their surface areas and sedimentation rates in water. The lowest-density TRWPs in the parking garage can be easily resuspended in the air and may be suspended in water for longer time.