Introduction
산업적으로 제조된 “liquid butadiene rubber (LqBR)” 는 고무산업에서 plasticizer, cure coagent, 그리고 타이어 트레드의 점탄성을 개선하는 용도로 사용된다. 특히, 타이어 산업에 적용하려는 연구가 지난 10년간 크게 증가하였는데, 이는 liquid polymer 관련 특허 출원 수의 큰 증가폭으로 확인할 수 있다.1
1995년부터 유럽의 processing oil 생산업체와 타이어 제조업체들은 기존에 사용하던 highly aromatic oil (distilled aromatic extract, DAE) 이 발암성 물질인 polycyclic aromatic hydrocarbons (PAHs) 을 함유하고 있어, 이를 대체하기 위한 가공조제를 개발하기 시작하였다. 실제로 2010년 EU REACH (Registration, Evaluation, Authorization and Restriction of Chemical) 규정에 따라 타이어 제조에서 DAE oils 의 사용이 금지되었고, treated distillate aromatic extract (TDAE) oil 과 같이 PAHs 함량이 적은 oil 이 대체 가공조제로 사용되고 있다.2,3 하지만 TDAE oil 이 적용된 가류물은 시간이 지남에 따라 TDAE oil 의 migration 이 발생하여 컴파운드 물성이 나빠지는 단점이 있다.4,5 이러한 문제들은 non-PAHs 이면서 migration 문제가 없는 새로운 가공조제에 대한 필요성을 제시하였다. 이에 따라 liquid polymer 에 대한 수요와 관심이 점차 증가하기 시작하였다.
처음 liquid polymer 는 레이싱 타이어 트레드 컴파운드의 점탄성을 개선하는 용도로 매우 요긴하게 사용되었다. 높은 vinyl 함량을 갖는 liquid polymer 는 base polymer 로 사용된 high vinyl styrene-butadiene rubber 와 상용성이 우수하여 컴파운드 Tg 를 증가시켰으며, 레이싱 타이어의 hysteresis 를 증가시켜 제동 성능을 극대화하였다.1 더 나아가 승용차용 타이어의 제조에서도 LqBR 을 가공조제로 적용하려는 다양한 시도와 함께 적용 기술이 점진적으로 발전하였다. 특히, 일본의 Kuraray Co. Ltd.에서는 non-functionalized LqBR의 vinyl 함량을 최소화하여 Tg 를 크게 낮추었고, solid polymer 와 co-vulcanization 시킴으로써 LqBR 의 migration 이 감소함을 확인하였다.6 Sumitomo Rubber Industries (Japan) 와 Continental AG (Germany) 는 윈터 타이어 컴파운드에 낮은 vinyl 함량의 LqBR 을 적용하여 저온에서의 viscoelastic performance 와 내마모성을 개선하였다.7,8 Hirata et al. 은 상업화된 non-functionalized liquid rubbers 를 가공조제로서 carbon black-filled NR 컴파운드에 적용하였고, 가공성 개선 및 migration 문제가 해결됨을 확인하였다.9 그러나 승용차 타이어 분야 내에서 LqBR 의 적용 영역을 넓혀 가기 위해서는 LqBR 의 free chain ends 로부터 기인하는 hysteresis 문제 해결이 필수적이었다.
Non-DAE plasticizers 에 대한 관심과 동시에, 1993년 Michelin 을 필두로 한 타이어 제조업체들은 온실가스 배출 저감을 위하여 기존의 카본블랙을 대체하여 실리카를 보강제로 사용하는 연구들을 진행하였다.10 소수성의 카본블랙과 달리, 실리카는 친수성의 표면 특성으로 인해 실란 커플링제를 사용하여 타이어 컴파운드의 traction 과 rolling resistance 를 개선하였으며, 이러한 filler technology 의 전환은 polymer 사슬에 functional group 을 도입하는 계기가 되었다.11,13
이러한 기술들을 기반으로 silane-functionalized LqBR 은 2017년부터 상용화 되었으며,14,15 타이어 컴파운드에 적용된 연구들이 보고되었다.16,18 Kim et al. 은 silane-functionalized LqBR 의 ethoxysilyl group 이 실리카의 silanol group 과 반응할 뿐만 아니라 가교제인 황에 의해 base polymer 와 crosslink reaction 을 함으로써 컴파운드 내에서 실란 커플링제와 유사한 역할을 수행할 수 있다고 보고하였다.19 게다가 silane-functionalized LqBR 로 processing oil 과 non-functionalized LqBR 을 대체할 경우, functional group 에 의한 chain mobility 감소와 free chain ends 수의 감소로 hysteresis 를 크게 감소시킬 수 있다. 또한, Hogan et al. 은 alkoxy, amino, cyano, sulfonyl, epoxy groups, halogen atoms 등으로 변성된 LqBR 이 실리카 표면에 존재하는 silanol group 과 화학적 결합을 형성할 수 있기 때문에 물성이 더욱 향상된다고 보고하였다.11,13
타이어의 성능이 polymer 의 거시구조(분자량, 분자량 분포, 고분자 곁가지 사슬 등)와 미세구조(styrene 함량, vinyl 함량 등)에 크게 의존하므로 타이어에서 요구되는 일련의 성능인자들을 만족시키기 위한 polymer 의 분자특성과의 관계를 이해하는 것은 대단히 중요하다. 그러나, 앞선 대부분의 연구에서는 직접 합성한 것이 아닌 상용화된 LqBR 을 사용하였고, 이에 따라 분자량, vinyl 함량, functionalization 조절에 있어서 LqBR 의 구조가 매우 한정적이었다. 이로 인해 LqBR 이 컴파운드 내에서 작용하는 메커니즘과 물성 결과에 대한 본질적인 이유를 설명하지 못하였다. 특히, LqBR 에 functional group 을 도입하는 합성법은 고도의 기술을 필요로 하며, 이렇게 합성된 functionalized LqBR (F-LqBR) 의 미세구조 (vinyl 함량) 에 대한 연구는 여전히 부족한 상황이다.
따라서, 본 연구에서는 음이온 중합법을 이용하여 분자량은 유사하고 vinyl 함량이 서로 다른 center-functionalized LqBR (C-LqBR, modified with ethoxysilyl group) 을 합성하였다. 그리고 이를 TDAE oil 을 대체할 가공조제로 적용하여 실리카 충진 고무 컴파운드를 제조하였으며, 타이어 트레드 컴파운드의 성능에 영향을 미치는 물성들을 평가하여 C-LqBR 의 vinyl 함량 효과를 확인하였다. 여기서 분석된 결과는 LqBR 이 적용된 타이어 트레드 컴파운드 제조에 필요한 최적의 LqBR 설계 및 선정의 기반을 마련해줄 것이라 기대된다.
Experimental
중합 시 사용한 물질들은 모두 질소 치환하였으며 유기용매로 cyclohexane (99%, Samchun Chemical Co., Seoul, South Korea), 음이온 개시제로서 n-butyl lithium (2.0 mol/L in cyclohexane, Sigma Aldrich Corp., Seoul, South Korea) 을 사용하였다. Monomer 로서 1,3-butadiene (Kumho Petrochemical Co., Daejeon, South Korea), vinyl 함량을 조절하기 위해 N,N,N′,N′-tetramethylethylenediamine (TMEDA, 99%, Sigma-Aldrich Corp., Seoul, South Korea) 을 polar modifier 로 사용하였다. 그리고 LqBR 을 변성시키기 위한 커플링제로서 tetraethyl orthosilicate (TEOS, 98%, Sigma-Aldrich Corp., Seoul, South Korea), 종결제로서 n-octyl alcohol (99%, Yakuri Pure Chemicals Co. Ltd., Kyoto, Japan) 을 사용하였다.
Solution styrene butadiene rubber (SSBR) 인 SOL-5220M (Kumho Petrochemical Co. Daejeon, South Korea, styrene content: 26.5 wt%, vinyl content: 26 wt%, non-oil extended) 과 high-cis butadiene rubber (CB24, Lanxess Chemical Industry Co., Ltd., Cologne, Germany; cis content: 96 wt%) 이 base polymer 로 사용되었고, 실리카(ZEOSIL 195MP, Solvay Silica Korea Co., Ltd., Gunsan, Korea) 가 filler 로 사용되었으며, X50-S (Evonik Industries AG, Essen, Germany; bis-[3-(triethoxysilyl)propyl]tetrasulfide (TESPT) 50%, carbon black N330 50%) 가 실란 커플링제로 사용되었다. 그리고 배합 가공 조제로서 treated distillate aromatic extracted (TDAE) oil (Kukdong Oil & Chemicals Co., Yangsan, Korea) 을 사용하였다. 활성화제로 ZnO 와 스테아르산(both from Sigma-Aldrich Corp., Seoul, Korea), 오존방지제로 N-(1,3-dimethylbutyl)-N′-phenyl-p-phenylenediamine (6PPD, Kumho Petrochemical Co., Daejeon, Korea) 이 컴파운드에 사용되었다. 그리고 가교제로 황(Daejung Chemicals & Metals Co., Siheung, Korea), 촉진제로 n-cyclohexyl benzothiazyl-2-sulfenamide (CBS, 98%, Tokyo Chemical Industry Co. Ltd., Tokyo, Japan) 와 1,3-diphenylguanidine (DPG, 98%, Tokyo Chemical Industry Co. Ltd., Tokyo, Japan) 가 사용되었다.
분자량과 분자량분포는 gel permeation chromatography (GPC, Shimadzu, Kyoto, Japan)를 이용하여 측정하였으며 GPC 는 solvent delivery unit, refractive index detector 와 3 종류의 Styragel column: HT 6E (10 μm, 7.8 mm × 300 mm), HMW 7 column (15-20 μm, 7.8 mm × 300 mm), HMW 6E column (15-20 μm 7.8 mm × 300 mm) 으로 구성되어 있고, polystyrene 표준 sample (EasiCal PS-1 standard, Agilent Technologies, Santa Clara USA) 을 사용하여 분자량 보정을 하였다.
핵자기 공명기(1H NMR; Varian, Unity Plus 300 spectrometer, Garden State Scientific, Morristown, NJ, USA)를 이용하여 C-LqBR 내의 vinyl 함량을 확인하였다. C-LqBR 은 deuterochloroform (CDCl3, Cambridge Isotope Laboratories, Inc., Andover, MA, USA) 을 용매로 하여 5 mm NMR tube 에 15 mg/mL 농도로 용해시켰다. 측정은 acquisition time 3s, relaxation time 2s, frequency 300 MHz, temperature 25°C 조건으로 64번 스캔하였다.
C-LqBRs 의 유리 전이 온도(Tg) 는 시차 주사 열량계(DSC-Q10, TA Instruments, New Castle, DE, USA)를 사용하여 측정하였다. 샘플(3-6 mg)에 대한 thermogram 은 질소 대기하에서 분당 10°C 의 가열 속도로 샘플을 -120°C에서 -20°C로 가열하여 얻었다.
Rubber processing analyzer (RPA2000, Alpha Technologies, Hudson, Ohio, USA) 를 이용하여 컴파운드의 filler-filler interaction 을 평가하였다. 미가류 컴파운드의 storage modulus (G′) 는 strain 0.28%-40% 범위에서 60°C 온도로 측정되었다. 낮은 strain 영역에서는 실리카 agglomerates 가 파괴되지 않아 G′ 값이 큰 반면, 높은 strain 영역에서는 agglomerates 가 파괴되어 G′ 값이 작아진다. ΔG′ (G′ at 0.28%-G′ at 40%) 값을 Payne effect 라고 하며, 이는 filler-filler interaction 의 정도를 의미한다.
Mooney viscometer (Vluchem IND Co., Seoul, Korea)는, ASTM D1646에 따라, 미가황 고무 컴파운드로 채워진 공간을 로터가 회전할 때의 토크를 측정하여 고무의 가공성을 평가하는 장치이다. 로터의 회전속도는 2 rpm, 온도는 100°C 로 설정하고 1분간의 예열 이후 4분간 로터를 회전시켜 토크값을 측정하였다.
컴파운드의 가황 특성은 moving die rheometer (MDR, Myung Ji Co., Seoul, Korea) 을 이용하여 진동 각도 ±1°, 온도 160°C 를 유지한 상태에서 30분 동안 측정하였으며, minimum torque (Tmin) 와 maximum torque (Tmax), 스코치 시간(t10) 그리고 최적 가황 시간(t90)을 측정하였다.
가교밀도는 가류물 내에 가교점의 수로 결정된다. 가교밀도가 증가하면 가교점 사이의 분자량이 감소하고, 가교점의 수가 증가한다. 팽윤 시험에서 가교밀도가 높을수록 가교된 고무 사슬 사이에 용매의 침투가 어려워 상대적으로 팽윤이 적다. 10 mm × 10 mm × 2 mm 크기의 가류물 시편 무게를 측정하고 tetrahydrofuran (THF, 99%, Samchun Chemical Co., Seoul, Korea) 와 n-hexane (95%, Samchun Chemical Co., Seoul, Korea) 에 각각 25°C 에서 1일간 침지 시켜 시편 내부의 유기 첨가물을 제거한다. 이어서 시편을 25°C 에서 1일간 건조시킨 후 중량을 측정하여 추출된 유기 첨가물의 질량분율을 결정한다. Total crosslink density 를 측정하기 위해 유기 첨가물이 제거된 시편의 중량을 측정한 후 상온에서 1일간 톨루엔 용매에서 침지 시키고 팽윤된 시편의 무게를 측정한다. 그리고 Flory-Rehner 식 (1) 과 Kraus 식 (4) 을 이용하여 vulcanizate structure 를 분석하였다.20,21
ν: crosslink density (mol/g)
Mc: average molecular weight between crosslink points (g/mol)
νr: the volume fraction of rubber in the swollen gel at equilibrium
Vs: the molar volume of solvent (cm3/mol)
ρ: the density of the rubber sample (g/cm3)
χ: the polymer-solvent interaction parameter in Equation (3)
wdry: the weight of dry sample
wfiller: the weight of filler in the dry sample
wswollen: the weight of the swollen sample
ρrubber: the density of the rubber
ρsolvent: the density of the solvent
ν0: the molar volume of solvent
ẟp: the solubility parameter of polymer
ẟs: the solubility parameter of solvent
vr0: the volume fraction of rubber in the swollen unfilled rubber
vr: the volume fraction of rubber in the swollen filled rubber
m: the slope which represents the filler-rubber interaction
φ: the volume fraction of filler
가류물의 기계적 특성(인장강도, 모듈러스, 파단 시 연신율) 은 universal testing machine (UTM, KSU-05M-C, KSU Co., Ansan, Korea) 을 이용하여 ATSM D 412에 따라 제작된 100 mm (길이) × 25 mm (너비) 의 아령형 시편을 이용하여 500 mm/min 의 속도로 측정하였다.
내마모성은 Deutsche Industrie Normen (DIN) 마모시험기를 이용하여 DIN 53516 에 따라 측정하였다. 시편은 직경 16 mm, 두께 8 mm 인 실린더형으로 제작하였고, 40±1 rpm 으로 회전하고 있는 원통형 드럼에 부착된 연마포의 표면에 5 N 의 load 를 가하여 시편을 40 m 주행 시키고 감소된 질량을 측정하였다.
컴파운드의 동적점탄 특성에서 temperature sweep은 strain-controlled rheometer (ARES-G2, TA Instrument, New Castle, DE, USA) 를 이용하여 strain 0.5%, 10 Hz frequency 에서 -60ºC 부터 70ºC 까지 storage modulus (G′), loss modulus (G″), tan ẟ 를 torsion mode 로 측정하였다. 그리고 strain sweep 은 dynamic material thermal spectrometer (DMTS, Eplexor 500N, GABO GmbH & Co. KG, Germany) 를 이용하여 temperature 60ºC, 10 Hz frequency 에서 dynamic strain 0.5% 부터 20% 까지 tension mode 로 측정하였다.
Center-functionalized liquid BR (C-LqBR) 의 중합 mechanism 은 Figure 1 에 나타내었다. C-LqBR 은 질소로 치환된 stainless-steel 반응기(2L) 를 이용하여 50°C 조건에서 음이온 중합으로 합성하였다. 이때 vinyl 함량이 서로 다른 C-LqBRs 을 합성하기 위해 TMEDA 의 양을 조절하였다.22 이후 1,3-butadiene 을 질소 압력을 이용해 반응기에 투입하였다. 모든 C-LqBRs 의 중합은 동일한 반응조건에서 이루어졌으며, 40분 동안 반응 시킨 후 BR 사슬 2개가 coupling 된 구조로 합성하기 위해 tetraethyl orthosilicate (TEOS, 0.5 molar ratio) 를 투입하여 반응을 종결시켰다.23 이후 vacuum evaporator 를 이용하여 C-LqBR solution 내 cyclohexane 을 증발시켜 C-LqBRs 을 수득하였다. C-LqBRs 의 거시구조와 미세구조는 GPC 와 1H NMR 을 이용하여 분석하였다.
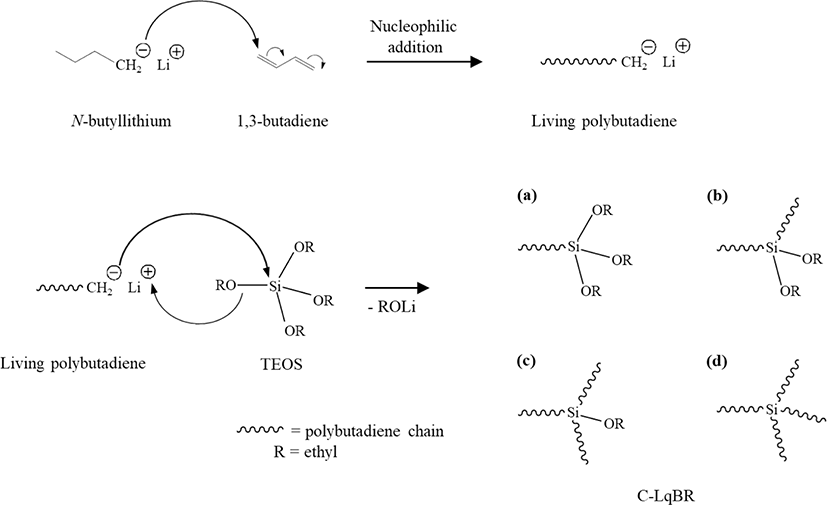
컴파운드는 Table 2 에 나타낸 배합표를 바탕으로 밀폐식 혼합기(300cc, Mirae Scientific Instruments Inc., Gwangju, Korea) 를 이용하여 제조하였다. Fill factor 는 혼합기 용량의 70%로 설정하였으며, 투입 단위는 parts per hundred rubber (phr) 로 고무를 기준으로 투여되었다. 가교구조 분석을 위해 100 phr의 실리카 함량을 갖는 컴파운드도 배합하였으며, 120 phr의 실리카 함량을 갖는 컴파운드의 물성 평가 결과만을 제시하였다. 배합 절차는 Table 3 에 나타내었고, 각 단계의 초기 온도는 100°C 와 50°C, 최종온도는 150-155°C 와 80-90°C 로 조절하였다. 각 단계의 배합이 끝난 후에는 two-roll mill 을 이용하여 컴파운드를 시트화 하였다. 이렇게 제조한 컴파운드는 moving die rheometer (MDR) 을 이용하여 160°C 에서 최적 가황시간을 확인한 후 160°C 의 유압프레스에서 최적 가황시간동안 가류하여 가류물을 제조하였다.
Results and Discussion
중합된 C-LqBRs 의 GPC 와 1H NMR 분석 결과를 Figure 2, 3 과 Table 4 에 나타내었다. Kim et al. 은 LqBR 의 분자량이 25,000 g/mol 이상일 경우, 대부분의 LqBR 이 황에 의해 base polymer 에 crosslink 될 수 있다고 보고한 바 있다.19 따라서, 본 연구에서는 C-LqBR 의 분자량을 25,000 g/mol 과 유사하게 합성하고자 하였다. GPC 측정 결과를 통해 C-LqBRs 이 21,000-25,000 g/mol 범위의 분자량 및 좁은 분자량 분포 (1.12-1.19) 를 갖는 것을 확인하였다. 그리고 NMR spectra 에서 butadiene 의 1,4-addition 구조는 5.37-5.50 ppm, 1,2-addition (vinyl) 구조는 5.50-5.60 와 4.79-4.99 ppm 에서 resonance peaks 를 나타내는데,24 면적의 비율을 계산한 결과 C-LqBRs 의 vinyl 함량이 9, 27, 51, 70 wt% 로 각각 합성되었다. Alkoxysilyl goup 에 결합된 ethoxy groups 의 1H chemical shift 는 1.19-1.26 ppm (SiO-CH2-CH3) 에서 triplet, 3.75-3.85 ppm (SiO-CH2-) 에서 quartet 을 나타내었다.25 C-LqBRs 의 coupling 된 polybutadiene 사슬의 개수를 나타내는 coupling number (CN) 는 coupling reaction 전후의 수 평균 분자량(Mn) 비율로 계산되었다.26
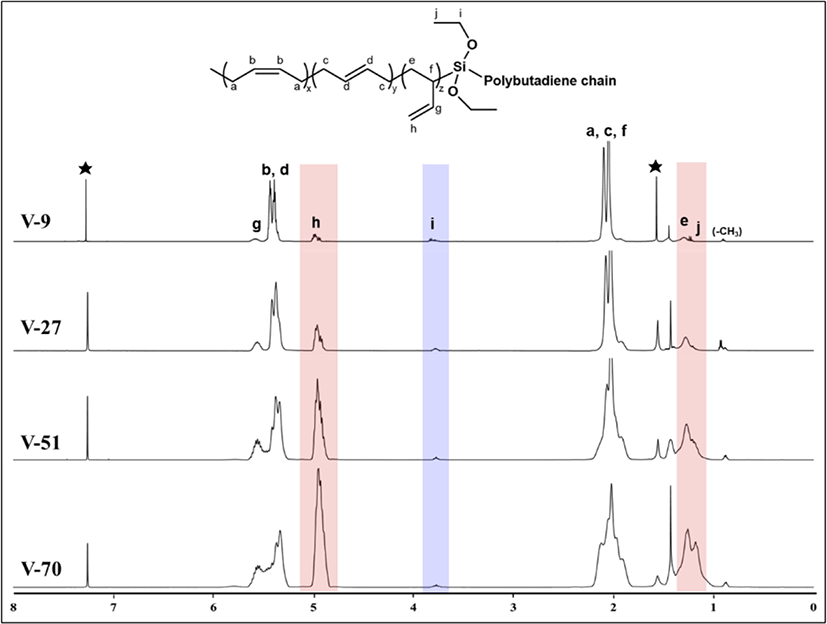
C-LqBRs 의 coupling number 가 1.9-2.3 값을 가지는데 이는 polybutadiene 사슬 2개 (Figure 1(b))가 coupling 되는 반응이 지배적임을 나타낸다.
1H NMR spectra 에서 각각의 H 에 대한 resonance peak 의 면적과 수소 원자 수는 비례한다. 이러한 원리에 따라, C-LqBR 사슬에 존재하는 ethoxy-silyl group 의 비율인 functionality 를 vinyl group 의 proton 과 alkoxysilyl group 의 proton peak 면적의 비율로 계산하였다.27
Svinyl-H 과 SAlkoxysilane-H 는 vinyl group 과 alkoxysilyl group 의 proton peak 면적을 나타낸다. RVinyl 은 vinyl 함량, Mn 은 C-LqBR 의 수평균 분자량, MB 는 1,3-butadiene monomer 의 분자량, nalkoxysilane 은 alkoxysilane 의 수소원자 개수, i.e., -Si-(OCH2CH3)2; “4” 를 나타낸다. F 는 변성률(Si/Chain), 다시 말해서; “1” 은 polybutadiene 사슬 2개가 coupling 되어 중심에 실리콘 원자 1개가 있음을 나타낸다.
Figure 4, Table 5 에 제시한 Payne effect 는 미가류물의 filler-filler interaction 을 나타낸다.28 그리고 strain amplitude 증가에 따른 storage modulus (G′) 의 감소는 filler network 가 파괴되어 나타나는 결과이며, △G′ 값이 클수록 강한 filler-filler interaction 을 나타낸다.
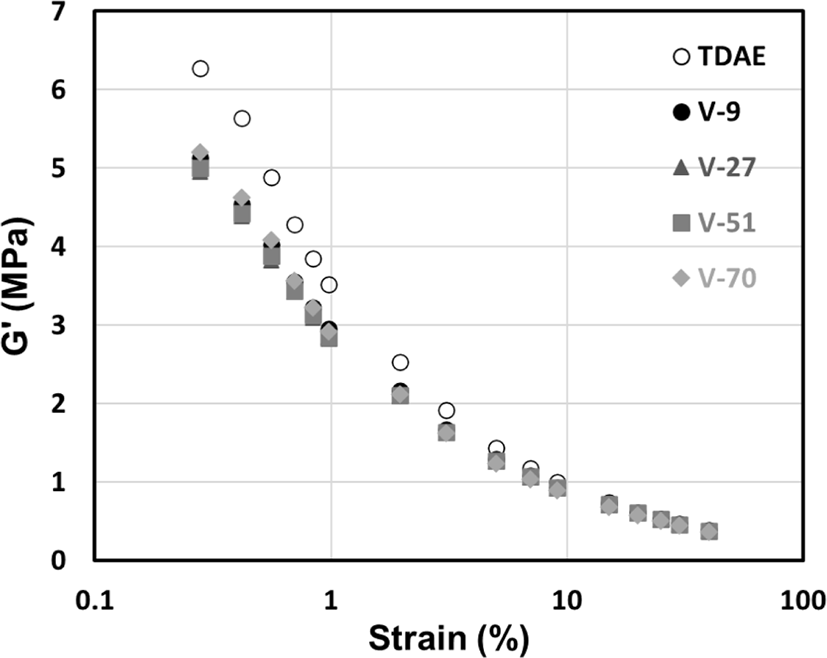
Compound | TDAE | V-9 | V-27 | V-51 | V-70 |
---|---|---|---|---|---|
△G′ (0.28-40%, MPa) | 5.9 | 4.74 | 4.57 | 4.62 | 4.84 |
C-LqBRs 은 ethoxy group 이 실리카 표면을 개질 시킴에 따라 실리카 분산이 향상되어 TDAE 컴파운드 대비 낮은 △G′ (G′ at 0.28%-G′ at 40%, MPa) 결과를 나타내었다. 반면, vinyl 함량에 따른 △G′ 값의 차이는 거의 없었기 때문에 실리카 분산의 경우, C-LqBR 의 functional group 의 효과가 지배적이라 판단된다.
컴파운드의 가공성과 관련이 있는 Mooney viscosity 와 moving die rheometer (MDR) 를 이용해 얻은 가황 특성 결과는 Figure 5 와 Table 6 에 나타내었다. C-LqBRs 을 적용한 컴파운드들의 Tmin 값은 TDAE 컴파운드보다 낮은 값을 나타내었다. 이는 Payne effect 결과에서 확인한 바와 같이 functional group 이 silanization reaction 을 통해 실리카 표면을 소수화 시킬 수 있어 실리카의 분산이 개선됨에 따라 나타난 결과라 판단된다. 그리고 C-LqBRs 은 실리카 분산을 개선시킬 뿐만 아니라 base polymer 사슬 사이에서 윤활유 역할을 하여 사슬 미끄러짐이 잘 일어남에 따라 C-LqBRs 를 적용한 컴파운드들이 TDAE 컴파운드보다 낮은 Mooney viscosity 값을 나타내었다.
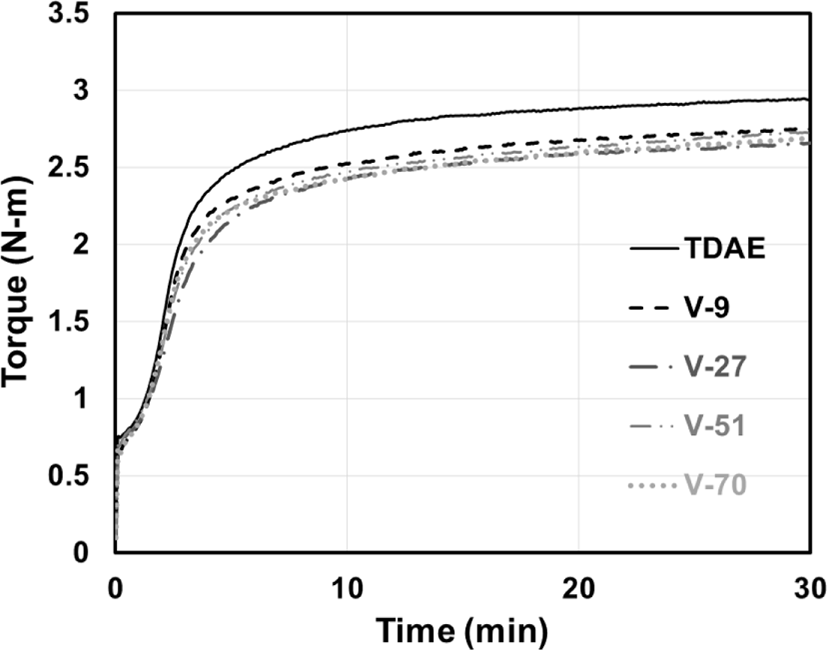
실리카 컴파운드의 경우, MDR curve 에서 델타 토크(ΔT; Tmax–Tmin) 값이 filler 의 morphology 와 total crosslink density 에 영향을 받는다고 알려져 있다.29 C-LqBRs 은 우수한 실리카 분산을 유도할 뿐 아니라 base polymer 사이의 가교 결합에 사용되는 황을 소모하기 때문에 C-LqBRs 를 적용한 컴파운드들의 ΔT 값이 TDAE 컴파운드에 비해 낮은 값을 나타내었다. 이러한 결과로 부터 total crosslink density 값 또한 TDAE 컴파운드 보다 낮을 것으로 예상된다.30
고무 컴파운드에 가교제로 황이 사용되었을 때, 가교 중에 황(S8)은 고리가 열리면서 고무의 allylic hydrogen 또는 이중결합과 반응하여 가황 반응이 일어난다.31 가교 반응의 active site 를 생성하는 allylic hydrogen 의 수가 많을수록 최적 가황 시간(t90)은 짧아지게 된다. BR 의 미세구조에 따른 allylic hydrogen atom 의 개수를 Figure 6 에 나타내었다. 그리고 C-LqBR 은 vinyl 함량이 증가함에 따라 C-LqBR 내에 존재하는 total allylic hydrogen 개수가 감소하여 t90 이 증가하는 경향을 나타내었다.
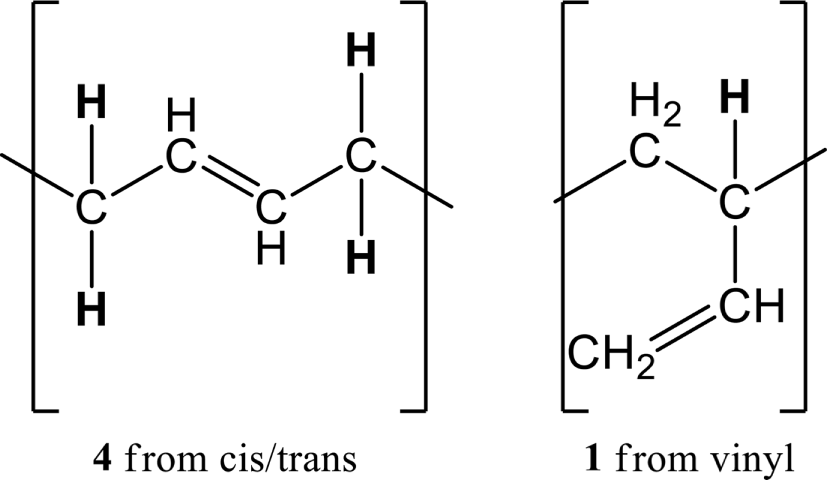
가류물 내에 존재하는 유기물의 양을 계산하기 위해 2종류의 유기용매를 이용하여 가류물 시편으로부터 유기물을 추출하였다. 먼저, 배합 시 투입된 TDAE oil 과 저분자량의 물질이 THF 에 의해 추출되었다. 이어서 THF 추출 후 얻어진 시편으로부터 n-hexane 을 이용하여 soluble 한 free LqBR 을 추출하였다. 2종류의 유기용매로부터 추출된 유기물의 총 추출량을 Figure 7(a)과 Table 7에 나타내었다.
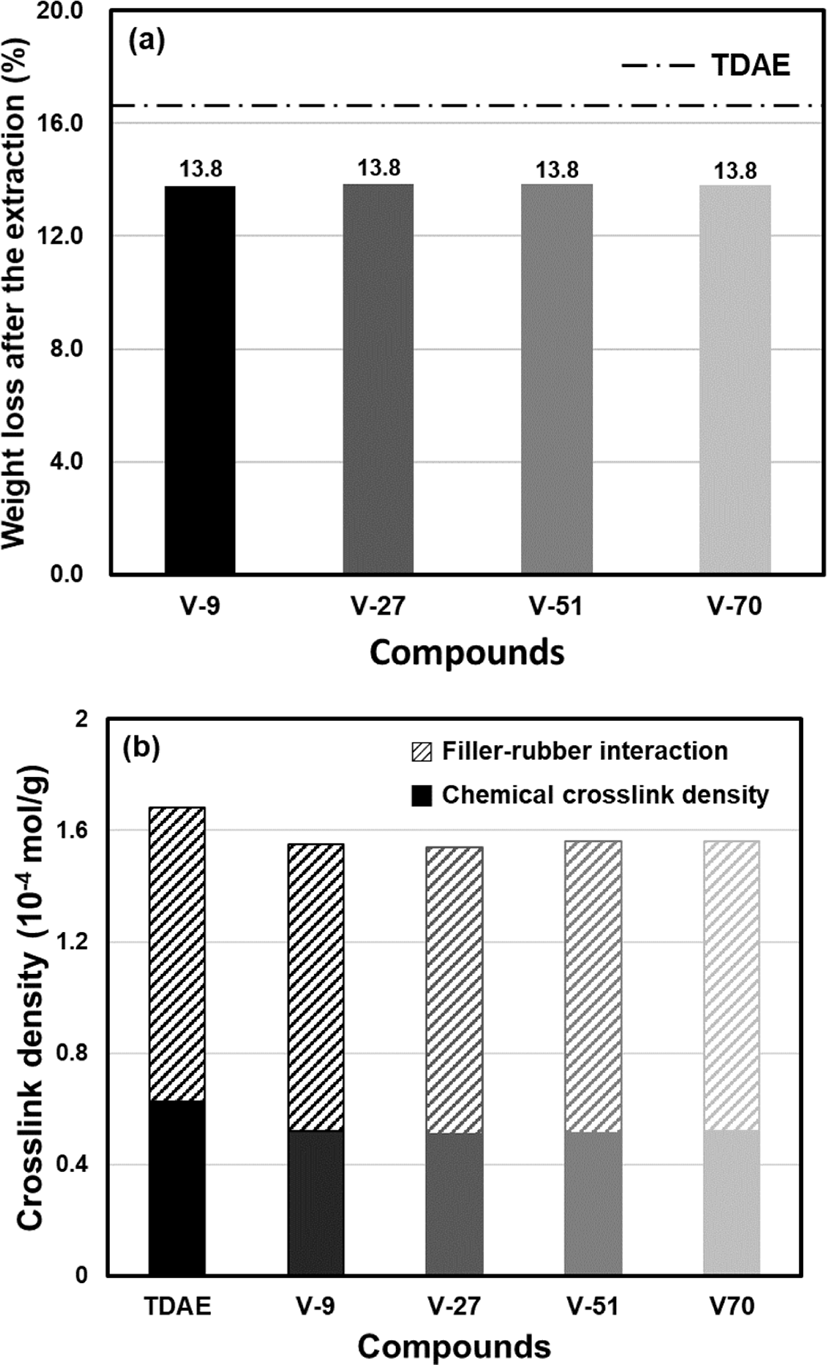
가류물 시편 내에서 TDAE oil 40 phr 의 비율은 13.6 wt% 이며, 그 중 10 phr 은 3.4 wt% 에 해당한다. TDAE 컴파운드의 유기물 추출량이 16.2 wt% (TDAE oil 40 phr; 13.6 wt% + 일부 첨가제; 2.6 wt%) 로 가장 높은 값을 나타내었는데, 이는 oil이 컴파운드 내에서 다른 재료와의 화학 결합을 형성하지 않아 유기용매에 쉽게 추출되기 때문이다. 반면 분자량이 25,000 g/mol 정도인 C-LqBRs 은 13.8 wt% (TDAE oil 30 phr; 10.2 wt% + 일부 C-LqBR; 1 wt% + 일부 첨가제; 2.6 wt%) 가 추출되어 70.6% 정도가 추출되지 않고 잔류하였는데 이는 functional group 이 silanization 반응을 통해 실리카 표면에 고정될 수 있고, 가류 시 base polymer 와의 co-vulcanization 을 통해 polymer network 에 고정될 수 있어 TDAE 컴파운드 대비 적은 추출량을 보였다. C-LqBR 의 vinyl 함량에 따른 유기물 추출량은 유사했으며, 이는 vinyl 함량에 관계없이 C-LqBRs 이 base polymer 에 co-vulcanization 할 수 있도록 각 컴파운드를 t90 동안 가류했기 때문이다(즉, C-LqBRs 추출량; 29.4%).
가교구조를 분석하기 위해 가류물의 total crosslink density 를 실리카-실란-고무 구조에 의한 filler-rubber interaction 과 황에 의한 chemical crosslink density 로 구분하였으며, 그 결과를 Figure 7(b)와 Table 7 에 나타내었다. 일반적으로 컴파운드 가류 시, 황은 base polymer 가교와 실란 커플링 반응에 각각 사용되어 chemical crosslink density 와 filler-rubber interaction 을 증가시킨다.15 변성되지 않은 LqBR 은 가류 시, 황을 소모하여 가류물의 chemical crosslink density 와 filler-rubber interaction 을 동시에 감소시켜 이 둘의 합인 total crosslink density 를 감소시킨다. 하지만 C-LqBR 은 functional group 이 실리카 표면에 고정될 뿐만 아니라 base polymer 와의 crosslink reaction 에 의한 화학적 결합을 형성하여 filler-rubber interaction 을 증가시킬 수 있다. 그 결과, vinyl 함량에 관계없이 모든 C-LqBR 컴파운드에서 TDAE 컴파운드 대비 chemical crosslink density 가 0.1×10−4 mol/g 이상 감소하고, filler-rubber interaction 은 유사한 값을 나타내었다.
기계적 물성과 DIN abrasion loss 측정 결과는 Figure 8 과 Table 8 에 나타내었다. Stress-strain curves 에서의 modulus at 300% elongation (M300) 값은 total crosslink density 결과와 높은 상관관계를 가진다고 알려져 있다.21 일반적으로 변성되지 않은 LqBR 은 base polymer chain 사이에서 윤활유 역할을 하여 미끄러짐이 잘 일어나게 하며, 황을 소모함으로 인해 total crosslink density 값이 감소하여 modulus 를 감소시킨다.19 그러나 C-LqBR 컴파운드는 TDAE 컴파운드 대비 total crosslink density 가 낮음에도 유사한 수준의 M300 값을 나타내었다. 이는 C-LqBR 이 coupling reaction 에 의해 TDAE 컴파운드 대비 유사한 filler-rubber interaction 을 가질 뿐만 아니라, C-LqBR 의 chain entanglement 영향으로 인해 modulus 값이 증가했기 때문이라 판단된다. 그리고 vinyl 함량에 따른 C-LqBR 컴파운드의 경우, 유사한 total crosslink density 와 vulcanizate structure 를 가지기 때문에 M300 값 역시 동등한 수준을 나타내었다.
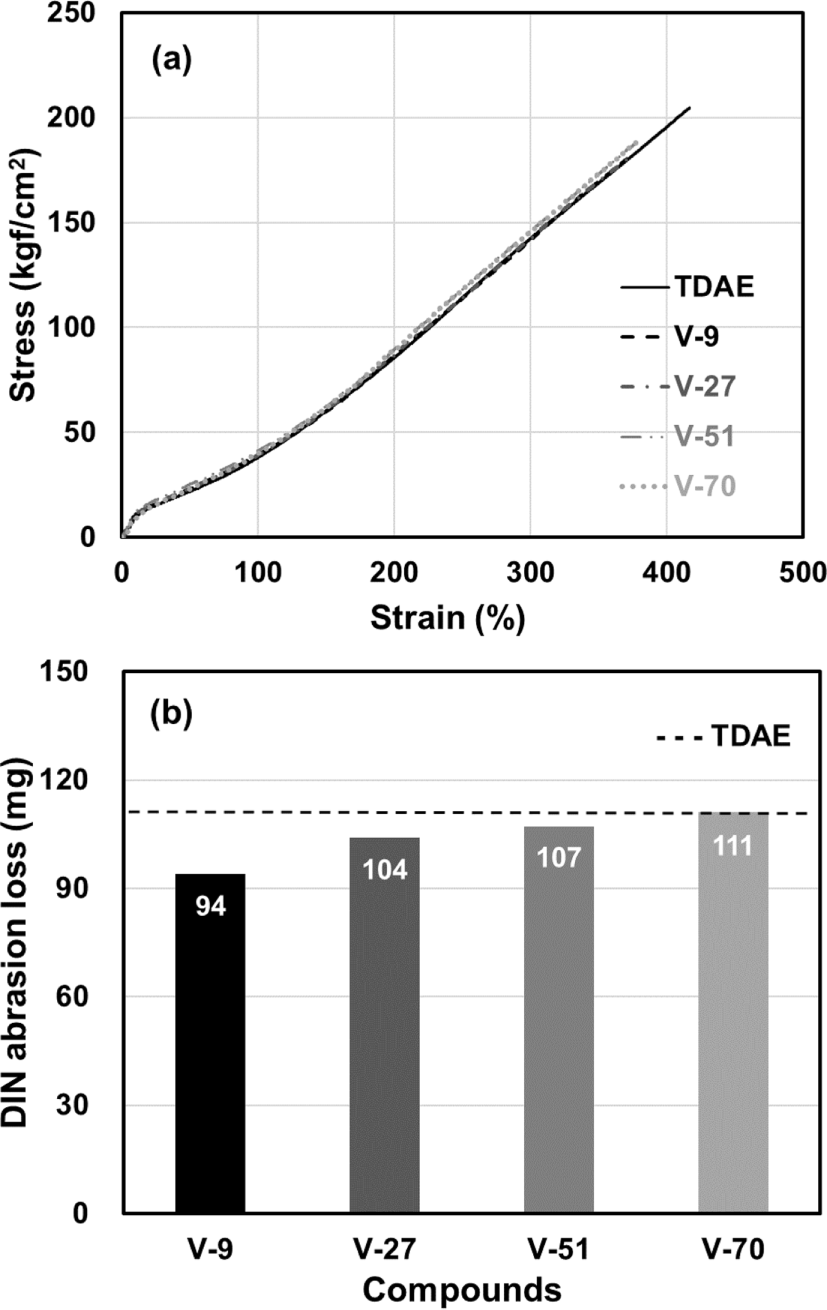
일반적으로 내마모성은 polymer 의 Tg 와 filler-rubber interaction 에 큰 영향을 받는다고 알려져 있다.32,35 TDAE oil보다 낮은 Tg 를 갖는 C-LqBRs 은 컴파운드의 Tg 를 소폭 낮추었으며, C-LqBR 의 vinyl 함량이 증가함에 따라 컴파운드의 Tg 역시 증가하는 경향을 나타내었다. 그 결과, V-9 컴파운드의 내마모성이 가장 우수하였고, V-70 컴파운드는 TDAE 와 유사한 내마모성을 나타내었다.
고무 컴파운드의 점탄성 특성은 타이어의 성능을 예측할 수 있는 실험실적 측정값이며, 둘 사이의 상관관계가 매우 높다. 그 중 -10°C 이하 저온 영역에서의 storage modulus (G′) 값은 snow traction 을 나타내는 지표로서, 값이 낮을수록 snow traction 이 향상된다고 알려져 있다.36,37 이는 저온 조건에서 낮은 G′ 값을 가질수록 타이어 트레드가 얼음 노면에서 쉽게 변형되고 adhesion 할 수 있기 때문이다.38 Loss modulus (G″) at 0°C 값은 타이어의 wet grip 성능을 나타내는 지표이며, 그 값이 높을수록 wet grip 성능이 우수해진다고 알려져 있다.39,40 그리고 G″ 값은 effective filler volume fraction 이 높을수록 높게 나타난다.41 Tan δ at 60°C 값은 타이어의 rolling resistance (RR) 를 나타내는 지표로서, 그 값이 낮을수록 연비 성능이 향상된다고 알려져 있다.42 이 온도에서의 main energy dissipation 은 filler-filler network 의 파괴 및 재생성에 기인한다고 알려져 있다.41 Kitamura 는 이 온도 영역에서의 hysteresis 특성이 polymer 의 free chain ends 에도 기인한다고 보고하였다.43 그리고 Salort 는 저분자량의 butadiene rubber 가 base polymer 에 crosslink 될 경우, dangling ends 로 인해 hysteresis 가 발생한다고 보고 하였다.4 두 연구 결과는 고온 영역에서의 에너지 손실은 polymer 의 free chain ends 도 중요인자 임을 잘 보여준다.
Figure 9 과 Table 9 에 TDAE oil 과 C-LqBRs 이 적용된 컴파운드의 동적 점탄특성 결과를 나타내었다. 낮은 Tg 를 갖는 C-LqBRs 은 컴파운드의 Tg 를 낮추고, 저온에서 컴파운드의 flexibility 를 증가시키기 때문에 TDAE 컴파운드보다 낮은 G′ at -30°C 값을 나타내었다. 하지만 C-LqBR 의 vinyl 함량이 증가함에 따라 컴파운드 Tg 역시 증가하여 G′ at -30°C 값이 증가하는 경향을 나타내었다.
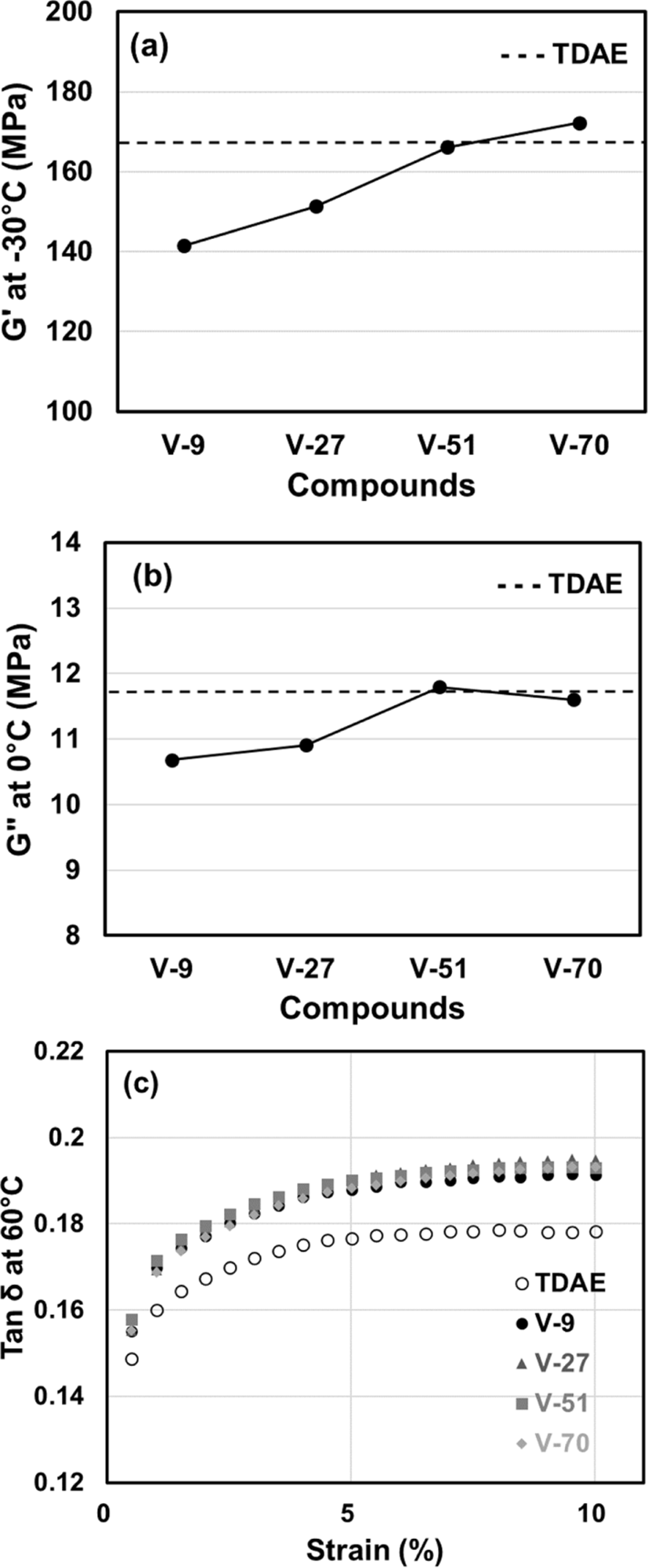
C-LqBR 컴파운드는 앞선 Payne effect 결과에서 확인한 바와 같이 실리카 분산이 향상됨에 따라 effective filler volume fraction 이 낮아져 TDAE 보다 낮은 G″ at 0°C 값을 나타내었다. 하지만 C-LqBR 의 vinyl 함량이 증가함에 따라 컴파운드 Tg 가 점차 증가하여 V-51 컴파운드부터는 TDAE 컴파운드와 유사한 G″ at 0°C 값을 나타내었다.
Figure 9(c)에 나타낸 바와 같이 C-LqBR 컴파운드들은 vinyl 함량에 관계없이 TDAE 컴파운드 대비 높은 tan δ at 60°C 값을 나타내었다. 이는 base polymer 에 결합하여 dangling 된 C-LqBRs 사슬이 free chain ends 로서 거동하여 hysteresis 를 유발했기 때문이라 판단된다. 그리고 컴파운드의 tan δ at 60°C 값 증가에는 C-LqBR 의 vinyl 함량보다 dangling ends 효과가 지배적이라 판단된다.
C-LqBR 의 vinyl 함량에 따른 컴파운드의 물성 변화의 경향을 Table 10 에 나타내었다. 이러한 C-LqBR 의 특성들은 저온조건에서의 high traction, long term flexibility 를 요구하는 윈터 타이어에 적합하다. Dangling chain ends 효과를 제거할 수 있는 양말단 functionalized LqBR 을 적용할 경우 tan δ at 60°C 의 값은 크게 개선될 것이다. 연구에서 제시된 결과는 LqBR 이 적용된 타이어 제조에 필요한 최적의 LqBR 설계 및 선정의 기반을 마련해줄 것이라 기대된다.
Types of processing aids | Payne Effect | Extraction Resistance | Abrasion Resistance | Tan δ at 60°C | G″ at 0°C | G′ at -30°C |
---|---|---|---|---|---|---|
TDAE oil | M | B | M | M | M | M |
9% | G | E | E | B | W | E |
27% | G | E | G | B | B | G |
51% | G | E | G | B | M | M |
70% | G | E | M | B | M | B |
Conclusions
본 연구에서는 silica filled rubber compounds 에 가공조제로서 적용한 C-LqBR 의 vinyl 함량이 물성에 미치는 영향을 확인하였다.
-
Functional group 을 갖는 C-LqBRs 은 기계적 물성의 하락 없이 Payne effect 를 개선시켰다. C-LqBR 은 vinyl 함량이 증가함에 따라 C-LqBR 내에 존재하는 total allylic hydrogen 개수가 감소하여 t90 이 증가하는 경향을 나타내었다.
-
C-LqBR 의 추출량은 TDAE oil 대비 크게 개선되었다. C-LqBR 은 가류 시, 황을 소모하여 TDAE 컴파운드보다 낮은 ΔT, total crosslink density 값을 나타내었다. 하지만 C-LqBRs 은 coupling reaction 을 통해 실리카와 base polymer 사이에 화학적 결합을 형성함에 따라 TDAE 컴파운드와 유사한 filler-rubber interaction 값을 나타내었다.
-
C-LqBR 의 vinyl 함량에 따른 컴파운드의 유기물 추출량과 mechanical properties 는 유사한 결과를 나타내었다. 반면, 내마모성은 vinyl 함량이 증가함에 따라 컴파운드의 Tg 가 증가하여 하락하였다.
-
Dynamic properties 는 C-LqBR 의 vinyl 함량이 증가함에 따라 컴파운드의 Tg 가 증가하여 snow traction 은 불리해졌으며 wet grip 은 개선되었다. Tan δ at 60°C 값의 경우, C-LqBRs 이 base polymer 에 crosslink 되면서 형성된 dangling ends 효과가 vinyl 함량보다 지배적임을 확인하였다.