Introduction
타이어의 구성요소 중에서 지면과 직접 접촉하는 부위를 트레드(tread)라고 한다. 트레드는 타이어에서 ‘magic triangle’ 성능이라 일컬어지는 내마모성(타이어 수명), 습윤제동(빗길 안정성), 구름저항(연비 특성)성능에 직접적인 영향을 미치므로 매우 중요한 역할을 한다.1,2 Magic triangle 특성들은 서로 간에 trade-off 관계에 있으므로 이를 동시에 개선하는 것이 트레드 컴파운드 개발에 있어서 핵심 요소이다. 일반적으로 트레드 컴파운드의 magic triangle 성능을 동시에 개선하기 위해서는 보강제인 silica의 분산성 향상과 filler-rubber inter-action (F-R interaction)의 향상이 필요하다.3-9일반적으로 타이어 트레드 컴파운드 배합에 사용되는 가공조제인 petroleum oil은 배합중에 컴파운드의 점도를 낮춰 가공성을 향상시킨다. 하지만, aging이 진행될수록 oil이 타이어 외부로 빠져나가는 migration 현상이 발생하고, 이로 인해 고무의 경도가 높아져 타이어 트레드의 변형율이 감소하게 된다. 그 결과로 타이어가 지면과 접촉하는 면적인 contact patch가 줄어들어 자동차의 제동 성능이 저하된다. 이는 가교물의 마모 성능이 감소하는 등 물성에도 악영향을 미친다는 단점이 있다.10,11 이러한 문제의 해결을 위해 migration 문제가 없는 새로운 가공조제에 대한 필요성이 증가하였다. 그 중에서 liquid butadiene rubber (LqBR)는 초기에 migration 현상을 해결할 용도로 사용되었으나, LqBR에 기능기를 부여할 경우 coupling agent의 역할을 수행하기 때문에 실리카 소수화를 통한 실리카 분산성 향상 및 기계적 물성, 내마모성 등의 개선 효과가 있다고 보고되었다.7,12-20
하지만 기능성 LqBR의 투입시기에 따라 실리카 표면의 실란올 그룹과 LqBR 및 실란 커플링제의 반응 순서가 달라지게 되면 filler-rubber interaction의 차이가 발생할 것으로 예상되어 LqBR의 투입 시기에 따른 트레드 컴파운드의 특성 거동 변화에 대한 연구가 필요한 상황이다.
따라서, 본 연구에서는 타이어 트레드 컴파운드에 투입되는 기존 가공조제인 TDAE oil 및 2종의 LqBR (non-funtional LqBR, silane terminated LqBR)을 이용하여 LqBR 사이의 투입 시기에 따른 컴파운드의 특성 변화를 분석하였다.
구체적으로는 컴파운드 미가류물 특성 평가인 bound rubber 함량, pyrolysis-GC 분석을 통한 bound rubber의 조성 비율, 가공성의 척도인 Mooney viscosity를 측정했으며, 가류물 특성 평가인 가황특성, 가교밀도, 유기물 추출비, 기계적 물성, 내마모성, 동적 점탄특성 분석을 실시했다. 이러한 연구 결과를 통해 non-functional LqBR 및 silane terminated LqBR과 같은 가공조제의 투입 시기를 최적화함으로써 LqBR적용 타이어 트레드 고무 배합 공정에 대한 가이드 라인을 제시하고자 한다.
Experimental
7종의 배합에 사용된 고무는 SSBR 5220M (Kumho Petro-chemical Co., South Korea, styrene content 26.5%, vinyl content 26%, non-oil extend) 이 사용되었다. 본 실험에 사용된 가공조제로는 TDAE oil, non-functional LqBR인 POLYVEST 110 (Evonik Industries, Germany, Vinyl content 1%, viscosity (20°C): 700-860 mPa·s)과 silane terminated LqBR인 POLYVEST EP ST-E60 (Evonik Industries, Germany, Vinyl content 22%, viscosity (20°C): 7,500-15,000 mPa·s)이 사용되었다.
실리카(Ultrasil 7000GR, Evonik Industries, Germany, BET: 175 m2/g)가 filler로 사용되었으며, bis[3-(triethoxysilyl)prop-yl]tetrasulfide (TESPT, Si-69)가 silane coupling agent로 사용되었다. 가황활성화제로 zinc oxide (ZnO), stearic acid (StA)가 사용되었으며, 산화방지제로 N-(1,3-dimethyl-butyl)-N′-phenyl-p-phenylenediamine (6PPD)가 사용되었다. 가교제는 normal sulfur가 사용되었으며, 가황촉진제는 diphenyl guanidine (DPG), n-cyclohexyl-2-benzothiazole sulfonamide (CBS)가 사용되었다.
배합 후 미가류 고무 컴파운드의 bound rubber 함량 분석을 위해 toluene (Samchun pure chemical, 99.8%), acetone (Samchun pure chemical, 99.7%)이 사용되었다. 가류물의 유기물 추출량 및 가교밀도 분석을 위해 tetrahydrofuran (THF, Samchun pure chemical, 99.8%), n-hexane (Samchun pure chemical, 95%)이 사용되었다.
컴파운드는 Table 1에 나타낸 배합표를 바탕으로 밀폐식 혼합기(kneader, 300cc)를 이용하여 제조하였다. 배합 순서는 Table 2에 나타내었고, dump temperature는 140~150°C, fill factor는 0.7로 설정하였다.
제조된 SMB 컴파운드는 50°C에서 2분간 밀폐식 혼합기를 이용하여 final masterbatch (FMB) 배합을 실시하였고, two roll mill을 이용해 시트화하였다. 이후 FMB는 가황특성 분석을 통해 구한 최적 가황 시간을 적용해 가열프레스에서 160°C 조건으로 가류물을 제조하였다.
배합이 완료된 후 미가류 컴파운드 특성 평가는 Mooney viscosity (ML1+4@100°C)와 bound rubber 함량 및 조성 측정, 그리고 Pyrolysis-GC 분석을 실시하였다. Mooney viscosity는 Mooney viscometer를 이용하여 ASTM D 1646에 따라 100°C 조건에서 1분간 예열한 뒤 4분동안 2 rpm의 속도로 회전시켜 값을 측정하였다. Bound rubber 평가는 티슈에 SMB 0.2g을 넣고, toluene 100 ml이 들어있는 vial에 6일간 보관하였다. 이때 3일째 되는 날에 toluene을 한 번 교체하였다. 그 뒤에 toluene의 세척을 위해 acetone으로 용매를 교체하여 24시간동안 보관한 후, 105°C 오븐에서 24시간 건조시켜 질량을 측정하였다. 이때 측정된 무게는 식 (1)에 대입하여 bound rubber의 함량을 계산하였다. Pyrolysis-GC (Py-GC) 분석용 샘플은 티슈에 SMB 0.2g을 넣고 toluene 100 ml이 들어있는 vial에 7일간 보관한 후, 105°C 오븐에서 24시간 건조시켜 준비하고 ref.20 논문에 기술되어 있는 분석 방법으로 Pyrolysis-GC 분석을 실시하였다. 구체적으로는 Py-GC 분석 시 발생하는 주요 열분해 생성물 4-Vinylcyclohexene 및 Styrene의 분석 결과를 Curve fitting equation에 대입하여 SBR과 BR의 성분비를 도출하였다.
RB : bound rubber content (%)
Wfg : the weight of the filler and gel
Wt : the weight of the sample before immersion
mf : the weight fraction of the filler in the compound
mr : the weight fraction of rubber in the compound
가류물의 특성 평가는 가황특성, 정량적 가교밀도, 유기물 추출비, 기계적 특성, 내마모성, 동적점탄특성(temperature sweep) 분석을 실시하였다.
가황특성은 FMB 시편을 oscillating disc rheometer (ODR) 장비를 이용하여 ±1°의 각도로 160°C 온도에서 30분동안 측정하였으며, 이를 통해 최대 토크 값(Tmax), 최적가황시간(t90)을 구하였다.
정량적 가교밀도 및 유기물 추출량 분석은 가류물을 10 mm × 10 mm × 2 mm의 시편 크기로 자르고 무게(A)를 측정하였다. 이후 가류물 시편의 유기물 제거를 위해 25°C에서 THF에 48시간, n-hexane에 24시간 침지시킨다. 이후 40°C에서 24시간동안 건조한 뒤 무게(B)를 측정하였다. 측정된 무게 값을 식 (2)에 대입하여 추출된 유기물의 질량비를 계산하였다.
건조가 완료된 시편을 toluene에 24시간동안 팽윤시키고, 팽윤된 시편의 무게(C)를 측정하였다. 측정된 무게는 식 (3)에 대입하여 vr 값을 계산하였다. 이후 계산된 vr 값을 식 (4, Flory-Rehner equation)에 대입하여 정량적 가교밀도를 구하였다.21-23,25
νr : the volume fraction of rubber in the swollen gel at equilibrium
Wd : the weight of the dried sample (B)
Wf : the weight of the filler in the sample
Ws : the weight of the swollen sample (C)
ρs : the density of solvent
ρr : the density of rubber.
ν : crosslink density (mol/g)
MC : average molecular weight between crosslink points (g/mol)
νr : the volume fraction of rubber in the swollen gel at equilibrium
Vs : the molar volume of solvent (cm3/mol)
ρr : the density of the rubber sample (g/cm3)
χ : the polymer-solvent interaction parameter (0.34)
기계적 특성(modulus, tensile strength, elongation at the break)은 ASTM D 412 조건에 따라 아령형 가류물 시편을 제조하여 측정하였다. 측정에는 universal testing machine (UTM, Daekyung T&T, Korea)가 사용되었으며, 500 mm/min의 속도로 시편의 기계적 특성을 측정하였다. 내마모성 측정은 DIN 마모시험기(Withlab, Korea)로 DIN 53516 규격에 따라 측정하였다. DIN 마모 시험 시편은 직경 16 mm 두께 8 mm의 실린더 형태의 시편으로 제조하였다. 연마포가 부착된 원통 모양의 DIN 마모 시험기를 이용하여 10N의 수직 하중 하에서 시편을 40m 마모시켜 시편의 질량 변화량을 측정하였다.
동적점탄특성 평가는 dynamic mechanical analyzer (DMA Q800, TA Instruments, USA)를 이용하여 temperature sweep 측정 조건으로 평가하였다. Temperature sweep 조건은 10Hz 조건 하에서 -80°C~70°C까지 3°C/min, 0.2% strain 조건으로 승온시키면서 가류물의 Tg, peak tan δ, tan δ at 60°C 값을 구하였다.
Results and Discussion
LqBR이 적용된 6종의 컴파운드의 가황특성을 Figure 1과 Table 3에 나타냈다. Oil이 투입된 T-1 컴파운드 대비 silane terminated LqBR이 투입된 T-5,6,7 컴파운드에서 높은 토크 값을 나타냈다. 이는 silane terminated LqBR이 실란 커플링제 역할을 하여 가교밀도가 증가하여 나타난 결과이다. Non-functional LqBR이 투입된 T-2,3,4 컴파운드 중 T-2 컴파운드에서 특히 높은 토크 값을 나타냈다. 일반적으로 실리카 컴파운드에서 maximum 토크 값(Tmax)은 가교밀도와 실리카 분산에 의해 영향을 받는다고 알려져있다.24-28 본 연구 결과에서 이후에 서술할 정량적 가교밀도 결과를 분석했을 때, 가교밀도는 유사한 결과를 나타내어 실리카 분산이 불리해 나타난 결과라 판단된다.
T-1~T-7 컴파운드의 Mooney viscosity 값과 bound rubber 측정 결과를 Figure 2과 Table 4에 나타냈다. Mooney viscosity 와 bound rubber는 유사한 경향을 나타냈다. 이는 높은 F-R interaction에 의해 bound rubber가 형성이 많아지고, bound rubber가 비교적 glassy한 특성을 나타내고 있기 때문에 나타난 결과이다. Bound rubber의 조성 분석을 위해 Py-GC 분석을 실시하였으며, GC Chromatogram을 Figure 3에 나타냈다. Py-GC 분석 결과를 Curve fitting equation에 대입하여 bound rubber의 조성을 분석한 결과, non-functional LqBR이 투입된 T-2,3,4 컴파운드의 경우에는 실란 커플링제가 먼저 투입될수록 bound rubber의 BR 조성이 증가하는 반면에, silane terminated LqBR이 투입된 T-5,6,7 컴파운드의 경우에는 silane terminated LqBR이 먼저 투입될수록 bound rubber의 BR 조성이 증가했다.
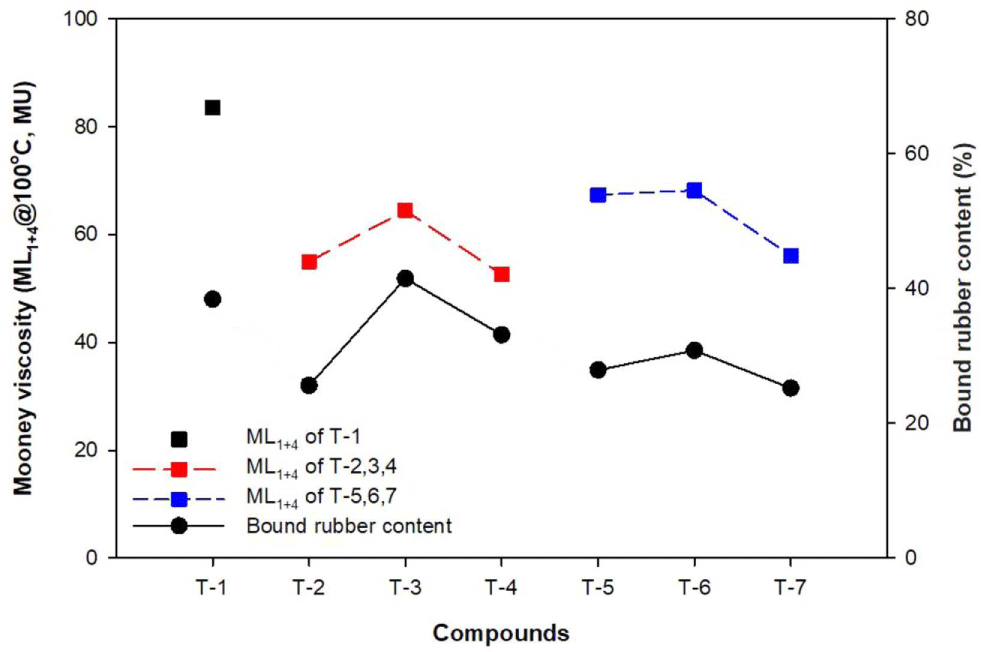
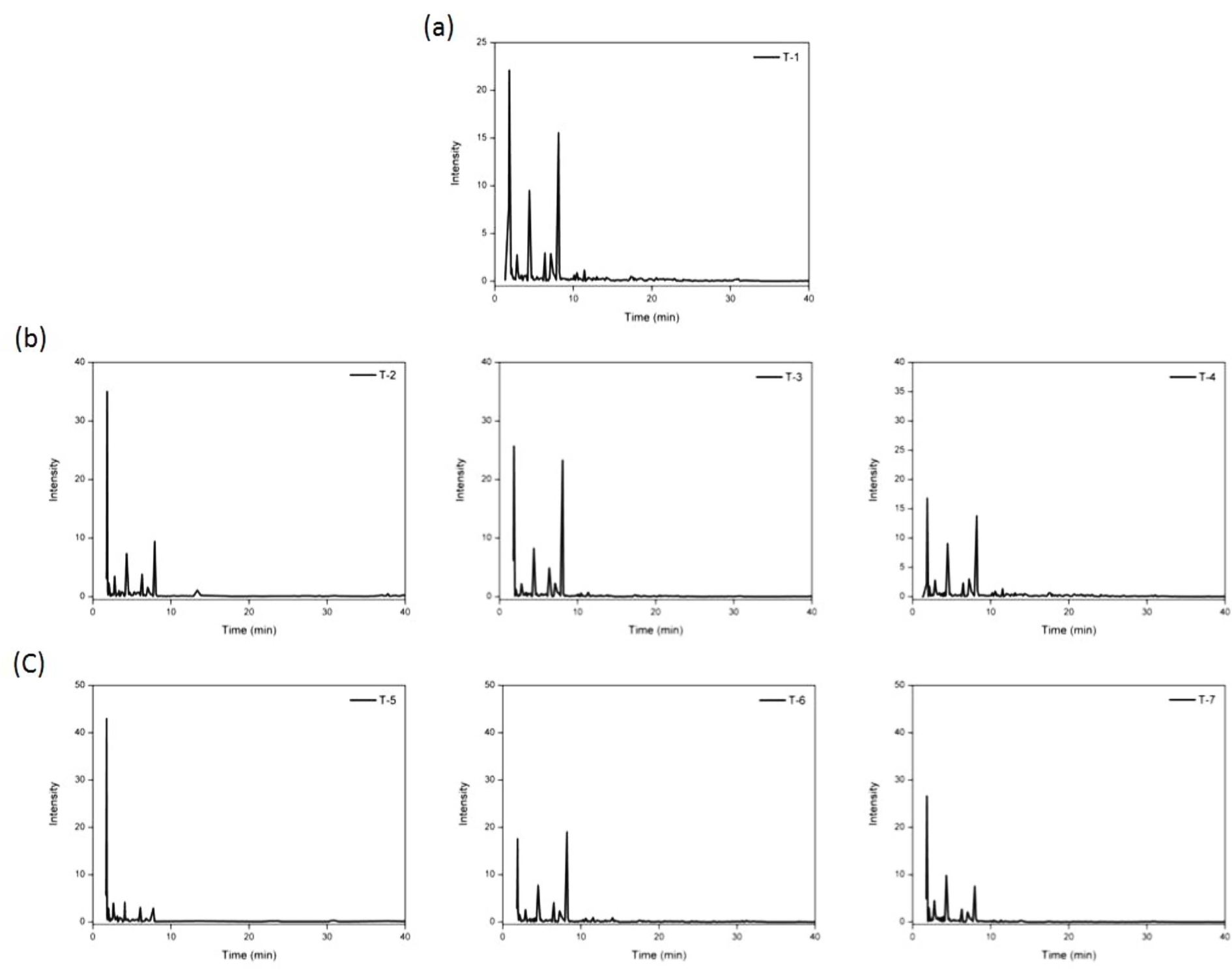
이는 실리카와 BR의 affinity에 의한 영향이다. 일반적으로 실리카와 BR은 affinity가 좋지 않다고 알려져 있다. Non-functional LqBR이 투입된 T-2,3,4 컴파운드의 경우 실란 커플링제가 먼저 투입되어 실리카 표면을 소수화 함에 따라서 점차 BR과의 affinity가 증가하고, 결과적으로 소수화도가 높아져 BR 조성이 증가하였다. Silane terminated LqBR이 투입된 T-5,6,7 컴파운드의 경우 silane terminated LqBR 또한 실리카 표면의 실라놀 그룹과 반응이 가능하고, 결과적으로 실란 커플링제보다 먼저 투입될수록 실라놀 그룹과 반응할 기회가 많아져 BR 조성이 증가하여 나타난 결과이다.
일반적으로 silane terminated LqBR이 투입되면 실리카 친화형 관능기의 영향으로 가류물에서의 F-R interaction이 증가한다. 하지만, bound rubber는 미가류물의 특성이며, silane terminated LqBR이 투입될 경우 관능기의 영향으로 실리카 표면을 소수화 함에 따라서 실리카 표면의 빈 공간으로 침투되어야 할 SBR chain이 차단되기 때문에 bound rubber의 함량이 감소하게 된다.
정량적 가교밀도 및 유기물 추출량 비율을 Figure 4와 Table 5에 나타냈다. 가류물을 THF, n-hexane에 침지시켜 제거된 유기물의 질량비를 비교하였다. Oil만 적용된 T-1 가류물이 가장 높은 유기물 추출비를 나타냈다. 이는 oil이 화학 결합을 형성하지 않기 때문에 유기 용매에 의해 쉽게 추출되기 때문이다. 또한, 투입된 TDAE oil 함량에 비해 낮게 나타난 유기물 추출량의 이유는, 투입된 10phr의 TDAE oil을 %로 환산했을 때 전체의 6.25%로 투입량 자체가 적기 때문에 나타난 결과이다. Non-functional LqBR이 적용된 T-2~T-4 가류물은 비교적 낮은 유기물 추출비를 나타냈으며, silane terminated LqBR이 적용된 T-5~T-7 가류물은 가장 낮은 유기물 추출비를 나타냈다.
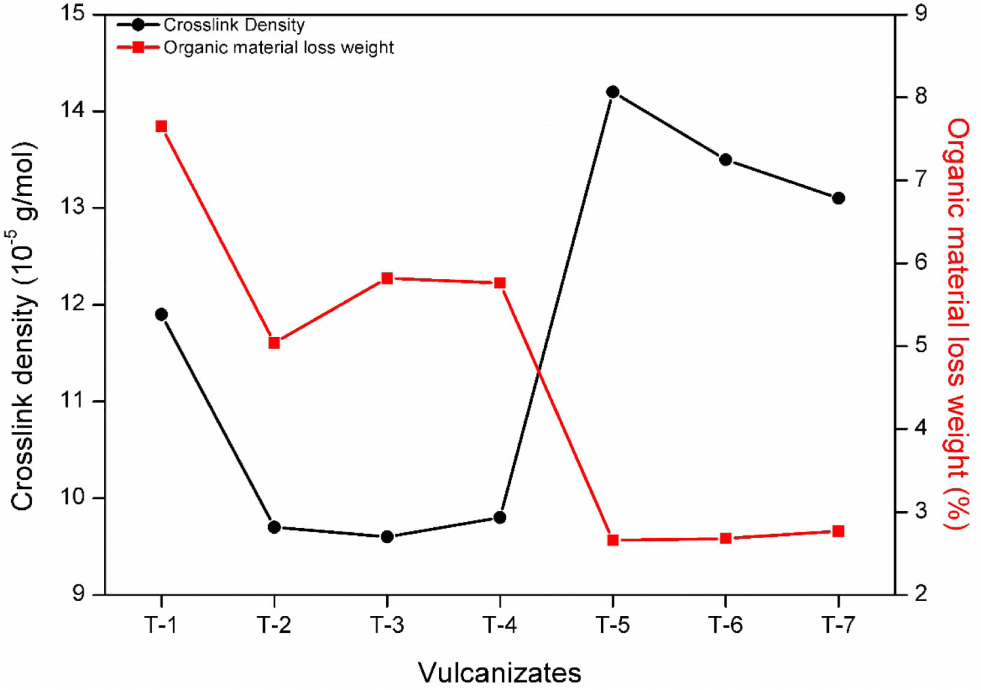
Unit | T-1 | T-2 (After) | T-3 (With) | T-4 (Before) | T-5 (After) | T-6 (With) | T-7 (Before) | |
---|---|---|---|---|---|---|---|---|
Crosslink density | 10-5 mol/g | 11.9 | 9.7 | 9.6 | 9.8 | 14.2 | 13.5 | 13.1 |
Organic matter loss | % | 7.65 | 5.04 | 5.82 | 5.76 | 2.66 | 2.68 | 2.77 |
Non-functional LqBR이 투입된 T-2,3,4 가류물에서는 free sulfur를 통해 SBR chain과 가교 결합을 형성하여 T-1 가류물 대비 비교적 감소한 유기물 추출량을 나타냈다. 또한, TDAE oil을 사용하지 않았음에도 상당량의 non-functional LqBR이 유기물로 추출된 이유는 분자량의 차이에서 기인한 결과이다. 일반적으로 가교점 사이의 분자량은 1만으로 알려져 있다. 하지만, non-functional LqBR의 분자량은 Mn 기준 약 2,600으로 분자량이 낮아 100% 가교에 참여하기는 어렵다. 따라서, 가교에 참여하지 못하고 가소제 역할을 수행한 non-functional LqBR이 추출된 것으로 판단된다. Silane terminated LqBR 역시 분자량이 약 3,200으로 낮지만, 양 말단의 작용기 때문에 가교 결합을 이루어 non-functional LqBR이 투입된 가류물 대비 낮은 유기물 추출량을 나타냈다.
Silane terminated LqBR이 투입된 T-5,6,7 가류물에서는 free sulfur를 통해 SBR chain과 가교 결합을 형성함과 동시에 실리카 표면의 실라놀 그룹과 실란화 반응이 가능하여 약 5%가량 가장 낮은 유기물 추출량을 나타냈다. 이는 실제 배합 설계에서 LqBR이 차지하는 weight %와 유사한 결과이다. 따라서, 이렇게 줄어든 5%는 silane terminated LqBR이 유기물로 추출되지 않았음을 확인할 수 있는 결과라고 판단된다. 또한 T-5,6,7 가류물 순서로 가교 밀도가 높은 이유는 실란 커플링제의 투입 시기에 따라 커플링 반응 정도의 차이로 인해 나타난 결과이다.
가류물의 기계적 특성 측정 결과를 Figure 5와 Table 6에 나타냈다. 300% modulus는 가교밀도와 높은 상관관계를 가진다고 알려져있다.25 우선적으로 non-functional LqBR보다 silane termianted LqBR이 투입된 가류물이 높은 가교밀도를 나타냈고, coupling reaction에 의해 TDAE oil이 투입된 T-1 가류물 대비 더 우수한 filler-rubber interaction을 가져 결과적으로 300% modulus도 우수한 결과를 나타냈다. Non-functional LqBR이 투입된 T-2~T-4 가류물은 LqBR이 sulfur의 소모에 의한 낮은 가교도가 원인이 되어 낮은 모듈러스를 나타냈지만 투입시기와 기계적 물성은 크게 차이가 없는 것으로 나타났다. 반면, T-5~T-7 가류물은 투입시기에 따라 기계적 물성에서 차이를 나타냈다. T-5, T-6, T-7 순서로 경도와 300% modulus가 높았고, 이는 LqBR의 투입시기에 대한 가교밀도의 차이로 판단된다. T-5 가류물의 경우 실란 커플링제와 LqBR이 차례로 반응하여 TESPT의 커플링 반응이 많이 일어나게 된다. 그 결과로 가교밀도가 높아지고 모듈러스 값이 높게 나타났다. T-6 가류물의 경우 실란 커플링제와 LqBR이 동시에 투입되어 T-5 가류물에 비해 TESPT의 커플링 반응이 적게 발생한다. 그 결과로 비교적 낮은 가교밀도를 이루어 모듈러스 값이 낮게 나타났다. T-7 가류물의 경우 실란 커플링제가 나중에 투입되어 T-5, T-6 가류물보다 TESPT의 커플링 반응이 적게 발생한다. 따라서, 가장 낮은 가교밀도를 이루어 모듈러스 값이 가장 낮게 나타났다.
각 가류물의 DIN 마모 손실량 결과를 Table 7에 나타냈다. DIN 마모 성능은 가교밀도, 실리카의 분산, F-R interaction 등에 의해 영향을 받는다고 알려져 있다.5,29,30 본 연구 결과에서도 실리카 가류물의 DIN 마모량은 앞선 기계적 특성 결과와 유사한 경향을 보였다. 측정 결과, Non-functional LqBR이 투입된 T-2~T-4 가류물의 DIN 마모량을 비교하면 투입 시기와는 무관하다는 것을 알 수 있다. 이는 동일한 가교밀도에 의한 결과이다. 반대로 silane terminated LqBR이 투입된 T-5~T-7 가류물의 DIN 마모량을 비교하면 투입시기에 따라 가교밀도의 차이로 인해 큰 편차를 나타냈다. Silane terminated LqBR이 투입된 T-5 가류물의 경우 실란 커플링제와 LqBR이 차례로 반응하고, T-6 가류물의 경우 실란 커플링제와 LqBR이 동시에 반응하고, T-7 가류물의 경우 실란 커플링제가 나중에 반응하였다. T-5, 6, 7의 순서로 TESPT의 커플링 반응 정도가 감소하여 가교밀도에 영향을 끼치고, 그 결과로 모듈러스 및 내마모성에 차이가 나타났다.
Unit | T-1 | T-2 (After) | T-3 (With) | T-4 (Before) | T-5 (After) | T-6 (With) | T-7 (Before) | |
---|---|---|---|---|---|---|---|---|
DIN abrasion loss | mm3 | 112.1 | 102.5 | 103.8 | 104.2 | 104.0 | 108.7 | 111.7 |
온도에 따른 가류물의 Tg, peak tan δ, tan δ at 60°C 값을 Figure 6와 Table 8에 나타냈다. Silane terminated LqBR이 투입된 T-5~T-7 가류물의 동적점탄특성을 비교하면 투입시기와 무관하다는 것을 알 수 있다. 반대로 non-functional LqBR이 투입된 T-2~T-4 가류물의 동적점탄특성을 비교하면 투입시기에 따라 편차를 나타냈다.
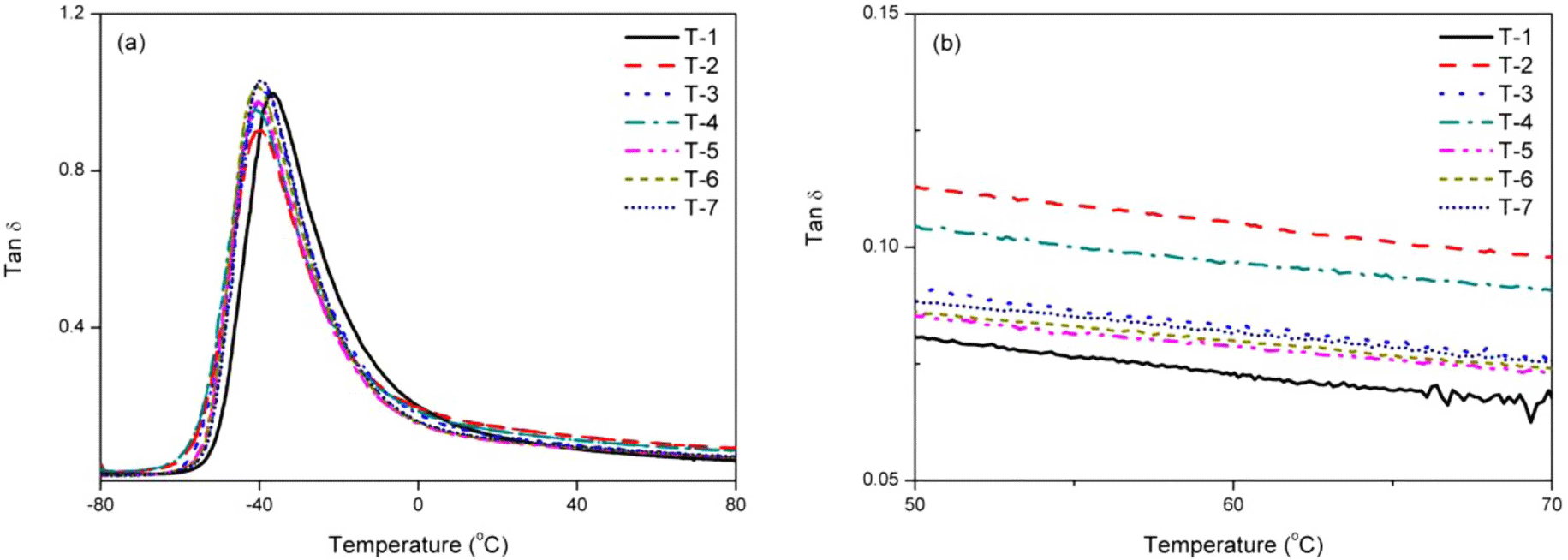
타이어 연비특성의 지표인 tan δ at 60°C은 가교 밀도가 높거나 실리카 분산이 우수할수록 낮은 값을 나타낸다고 알려져있다.11,21 Non-functional LqBR이 투입된 T-2,3,4 가류물의 경우 가교 밀도가 유사하게 나타났다. 따라서 동적점탄특성 측정 결과에서 값의 차이는 실리카 분산에 의해 나타난 결과이며, T-2,3,4 가류물 중 T-3 가류물의 분산이 우수하다고 판단된다. Silane terminated LqBR이 투입된 T-5,6,7 가류물의 경우 60°C에서 유사한 tan δ 값을 나타냈다. 하지만 앞서 측정한 가교 밀도 측정 결과를 봤을 때, 가교 밀도의 차이는 분명히 존재한다. 그럼에도 불구하고 60°C에서 유사한 tan δ 값의 차이는 실리카 분산에 의해 나타난 결과이며, T-5,6,7 가류물 중 T-7 가류물의 분산이 우수하다고 판단된다.
가류물의 Tg는 일반적으로 사용된 raw polymer의 Tg에 의해 영향을 받는다고 알려져있다. 이를 통해 알 수 있는 T-1 가류물 대비 나머지 가류물의 Tg 감소 원인으로는 LqBR의 Tg 자체가 POLYVEST 110 (Tg: approx. -100°C), POLYVEST ST-E60 (Tg: approx. -80°C)로 raw polymer의 Tg가 낮기 때문이다.
Peak tan δ값은 T-2 가류물을 제외한 나머지 가류물에서 비슷한 결과를 나타냈다. T-2 가류물의 경우 가교밀도는 동일한데 분산이 불리하여 Tg에서의 peak tan δ 값이 낮음을 알 수 있다.
Conclusions
본 연구에서는 타이어 트레드 컴파운드의 migration 문제 해결을 위해 가공조제로서 적용한 silane terminated LqBR이 물성에 미치는 영향을 확인하였다. 기존 타이어 트레드 컴파운드에 투입되는 가공조제인 TDAE oil 및 2종의 LqBR (non-funtional LqBR, silane terminated LqBR)을 이용하여 LqBR 사이의 투입 시기에 따른 컴파운드의 특성 변화를 분석하였다.
평가 결과, non-functional LqBR인 POLYVEST110이 투입된 고무 컴파운드는 실란 커플링제와 함께 투입하는 mixing procedure인 T-3에서 연비특성 지표인 tan δ at 60°C 값이 5% 이상 낮은 값을 나타내어 우수한 연비특성을 나타냈다. Silane terminated LqBR인 POLYVEST ST-E60이 투입된 고무 컴파운드는 실란 커플링제를 먼저 투입하고 이후에 투입하는 mixing procedure인 T-5에서 우수한 modulus 및 내마모성을 나타냈다. 또한, 투입 시기에 따라 bound rubber의 조성을 분석해 가류물 특성과 correlation 시켰으며, 유기물 추출량이 개선된 것을 확인하였다.