Introduction
Polyurethane (PU) is a versatile material used extensively in diverse industries. Its uses span from coating, adhesive, sealants, elastomers, to rigid and flexible foams. Rigid PU foams, recognized for their closed cells, low thermal conductivity, and high compressive strength, serve as excellent insulation materials. On the other hand, flexible PU foams with their open cells find wide usage in flexible applications like subway seats, mattresses, etc.1,2 However, polyurethane foams (PUF) are heat-sensitive and release harmful gases during combustion, posing limitations to their use.3,4
In order to solve these drawbacks, we incorporated polydimethylsiloxane (PDMS) into PUF to become polyurethane- hybrid foams. During combustion, the Si-O in PDMS transforms into SiO2, creating a char layer that obstructs atmospheric oxygen and slows down combustion.5 Therefore, hybridizing these two materials combines the benefits of PUF and PDMS. Additionally, under isocyanate and tin catalysts, PDMS can be foamed through a hydrogen condensation reaction, allowing it to foam alongside PU.
PU-PDMS hybrid foams, owing to hybridization, displays an increased density and cell size along with enhanced flame retardancy. In PUF, blowing agents are commonly used to control density and cell size among various physical properties. These blowing agents can be divided into chemical blowing agents (CBAs) and physical blowing agents (PBAs).6,8 CBAs such as water react with isocyanate to produce CO2 gas whereas PBAs dissolve in polyol and isocyanate at low temperatures and pressures, typically at room temperature and atmospheric pressure, and generate gas through evaporation. An increase in the temperature of solution due to the exothermic reaction decreases the solubility of PBA, causing it to change into a gaseous state and leading to the nucleation and growth of bubbles in the solution.7-9 Chlorofluorocarbons (CFCs) were used until the late 1950s and hydro-chlorofluorocarbons (HCFCs) until the early 1990s, but due to the issue of ozone layer depletion, they have been recently replaced by hydrocarbons (HC). HC, an eco-friendly PBA, has zero ozone depletion potential (ODP) and low global warming potential (GWP), coupled with a low cost, making it a suitable alternative foaming agent.7,10
In addition, thermal expandable microspheres (TEM), a non-traditional foaming agent, is being newly applied in polymeric foams. TEM encapsulates low-boiling liquid HCs into a thermoplastic polymer shell, and two transformations occur when the temperature rises: the liquid vaporizes and the shell simultaneously softens.11,12 As a result, the softened shell expands due to the pressure of the internal gas, increasing its size by 50 to 100 times. TEM separates the expansion mechanism from the curing reaction and phase separation does not occur unlike liquid PBA, simplifying the foaming process.13 Moreover, it has benefits such as low density, wide applicable temperature range, good solvent resistance, and low cost, making it versatile in applications including shoe soles, wallpaper, leather, etc.
In this study, we introduced various PBAs to PU-PDMS hybrid foams and investigated the changes in physical properties of the prepared foams. The addition of PBA is expected to significantly alter cell morphology, which in turn is likely to affect physical properties such as density and thermal conductivity. Therefore, the cell size, apparent density, and thermal conductivity of the foam were measured according to the type and content of PBA.
Experimental
All the chemicals employed in this research were used as provided. The PDMS functionalized with vinyl, OH and H was supplied by Korea Bio-Gen Co., LTD (South Korea). A two-component mixture (Flex-Foam iT, grades III) for making flexible polyurethane foam was obtained from Smooth-On, Inc. (USA). SUT-9 (28%, stannous octoate) was supplied by SD Korea (South Korea). Cyclohexane (99.5%) and hexane (95%) were purchased from Sigma-Aldrich (USA). TEM was provided by Dongjin Semichem CO., Ltd. (South Korea). The particle size of TEM used in the experiment is 15–25°C and the expansion temperature ranges from about 90°C to 120°C. The specific attributes of the blowing agents are enlisted in Table 1 and 2.
Substance | n-Hexane | Cyclohexane |
---|---|---|
Molecular weight (g/mol) | 86.18 | 84.16 |
Boiling point (°C) | 69 | 80.7 |
Viscosity (25°C, Cp) | 0.31 | 1 |
ODP | 0 | 0 |
GWP | low | low |
Substance | TEM |
---|---|
Average particle size (μm) | 15±5 / 25±5 |
Expansion start point (°C) | 90±5 |
Maximum temperature (°C) | 120±5 |
Figure 1 illustrates the preparation process for the PU-PDMS hybrid foam. The formulations for fabricating hybrid foams are detailed in Table 3. The initial process involves placing PDMS (excluding the H-polymer), polyol, catalyst, and various types of blowing agents into a paper cup, followed by a minute of mixing. Subsequently, the H-polymer and isocyanate were introduced into the pre-mixed components and stirred for an additional 30 s. The resulting mixture was then transferred into a 100 mm × 100 mm × 50 mm acrylic mold and cured inside an oven pre-set at 100°C for 20 min.
Resin | phr | |
---|---|---|
PDMS | Vinyl polymer | 13 |
OH polymer | 27 | |
H polymer | 2 | |
Polyol | 37 | |
Catalyst | 0.04 | |
Blowing agents | Hydrocarbon | 0.2-1 |
TEM | 1-3 | |
Isocyanate | 21 |
A scanning electron microscope (SEM, Hitachi S-4300, Japan) with an acceleration voltage of 15.0 kV was utilized to examine the microstructure of both the TEM and hybrid foams. The HC content encapsulated in TEM was assessed via thermogravimetric analysis (TGA, TGA 4000, PerkinElmer, USA). All samples were trimmed to a size of 75 mm × 75 mm × 25 mm and three specimens were prepared to calculate the apparent density. The chemical properties of the TEM and the synthesized hybrid foams were determined using fourier-transform infrared (FTIR) spectroscopy, equipped with attenuated total reflectance (ATR; Spectrum Two, PerkinElmer, USA). The thermal conductivities of the hybrid foams were measured using a HotDisk TPS2500 thermal constant analyzer (Therm Test Inc., Sweden). The software for image analysis (Image J) was employed to evaluate the pore sizes, and the foam structure was inspected using an optical microscope (DM2700 M, Leica, Germany).
Results and Discussion
First, the expansion behavior of TEM was assessed at 100°C by comparing SEM images of microspheres at room temperature (Figure 2(a)) and after 20 minutes of expansion at 100°C (Figure 2(b)). As indicated in Table 2, the initial diameter averaged at 20μm and it expanded up to 100μm. The slightly distorted appearance is likely a result of the vacuum state during the SEM measurement. To ascertain the quantity of the internal blowing agent, TGA analysis was performed as illustrated in Figure 3. Weight changes started manifesting from 100 to 130°C due to the evaporation of an internal blowing agent. The weight loss about 20 wt% is considered as the amount of the blowing agents in the microspheres. The weight loss occurring above temperatures of 350°C is presumably due to decomposition of acrylonitrile copolymer shell of the microspheres.
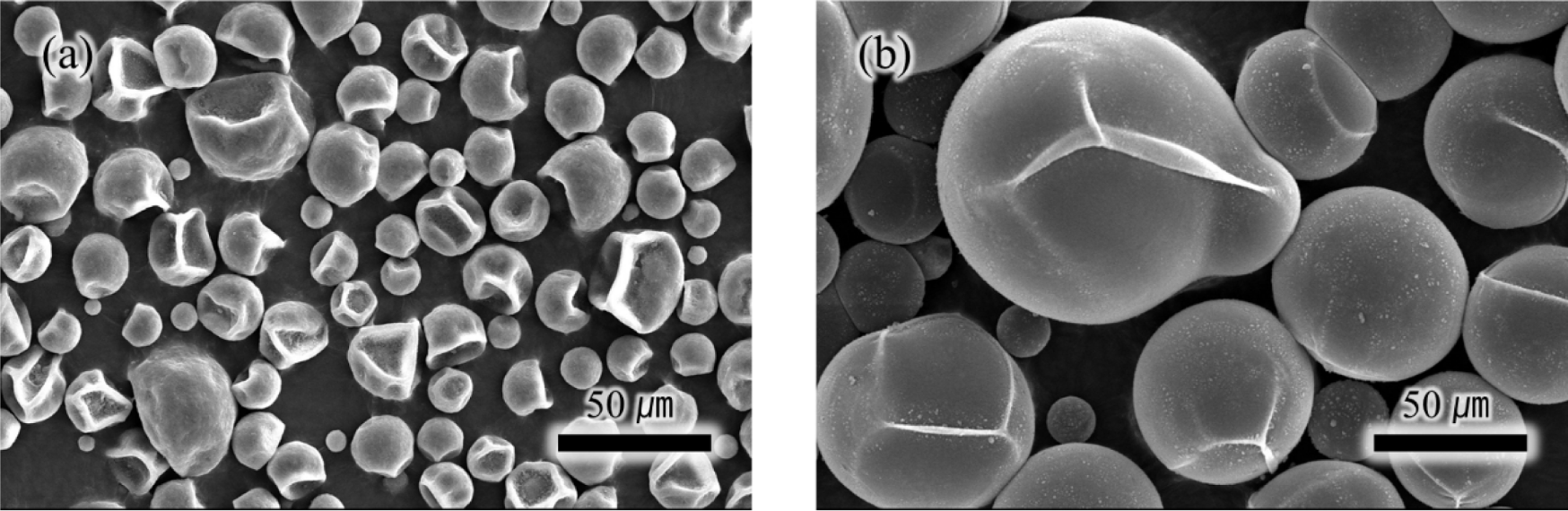
PU-PDMS hybrid foams with hydrocarbon-based PBA were prepared with different content (0.2, 0.5, 1 phr). When more than 1 phr was added, the foam began to develop large internal voids, leading to structural collapse. Figure 4 shows images of the as-prepared PU-PDMS hybrid foam with hydrocarbon-based PBA immediately after the foaming process and cut foams to measure its apparent density. The addition of hydrocarbon-based PBA did not visibly alter the appearance when compared to the neat foam. Moreover, it appeared that the height of the foam was reduced. On the other hand, when TEM was added, the foam exhibited a larger volume compared to the neat foam as seen in Figure 5. Additionally, it can be observed that as the content increased of PBA, the height of the foam also increased. It seemed that the height had increased as the thermal expansion capsule expanded within the foam matrix. It is noted that TEM was evenly distributed inside foams, contributing to the overall height increase. Unlike the hydrocarbon-based PBA that generated large bubbles in the foaming process, TEM results in uniformly sized cells in foams.
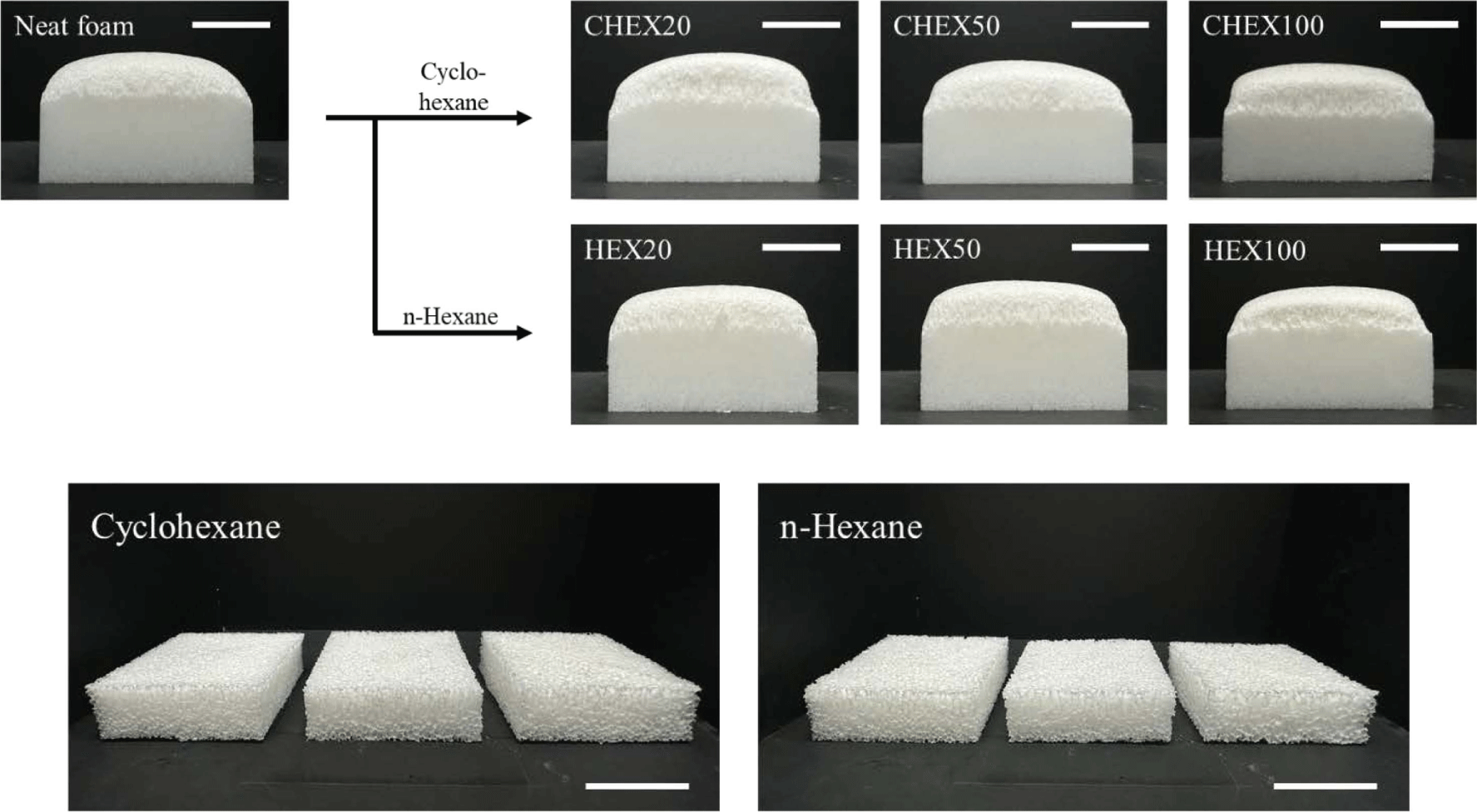
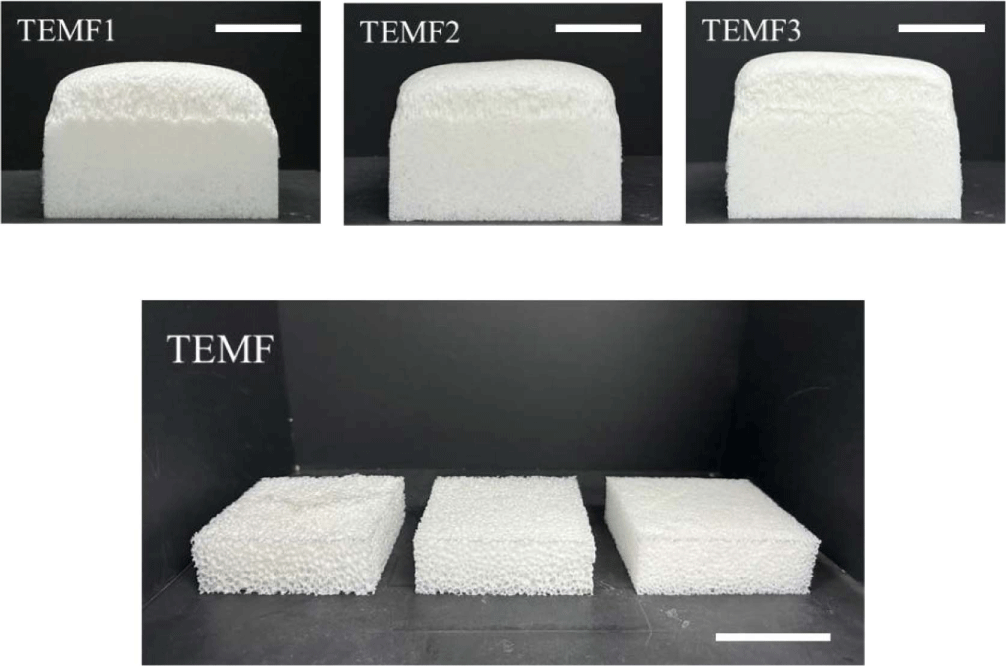
FTIR spectra of foams are shown in Figure 6. The C-H stretching in CH3 and CH3 symmetric bending in Si-CH-3- are observed at 2964 cm−1 and 1261 cm−1. Peaks at 1088 cm−1 and 1015 cm−1 are related to Si-O-Si stretching vibrations, and the peak at 796 cm−1 is attributed to Si-C stretching and CH3 rocking vibrations. These distinct peaks reveals that PDMS is well incorporated in the hybrid foams.
Additionally, peaks at 1733 cm−1, 1540 cm−1 and 1511 cm−1 are caused by C=O stretching vibration of urethane, N-H bending vibration of urethane and bending vibration of disubstituted urea, respectively.15,16 Therefore, it can be seen that PU and PDMS exist in the foam structure.
Figure 7 shows the hierarchical pore structure of neat PU-PDMS hybrid foam without any PBA, which is generated by different foaming reactions. It is noted that the fabrication of next PU-PDMS hybrid foam was conducted without PBA and other procedures were identical. PU-PDMS hybrid foam undergoes two types of foaming reactions. For PU, CO2 gas is produced when water acting as a chemical blowing agent reacts with isocyanate. Additionally, H2 gas is generated by condensation reaction between H-PDMS and vinyl-PDMS for PDMS. The hierarchical pore structure by CO2 and H2 gas is visible through optical microscope and SEM, respectively. Large cells generated by CO2 are surrounded by small cells generated by H2 gas. And the cells are evenly spaced throughout the PU-PDMS hybrid matrix. This evenly distribution of pores suggests that the foaming reaction of PU and the condensation reaction of PDMS occur uniformly at a balanced speed for two different blowing mechanisms. If the blowing speed for each mechanism is different, this uniform distribution of cells cannot not be observed.
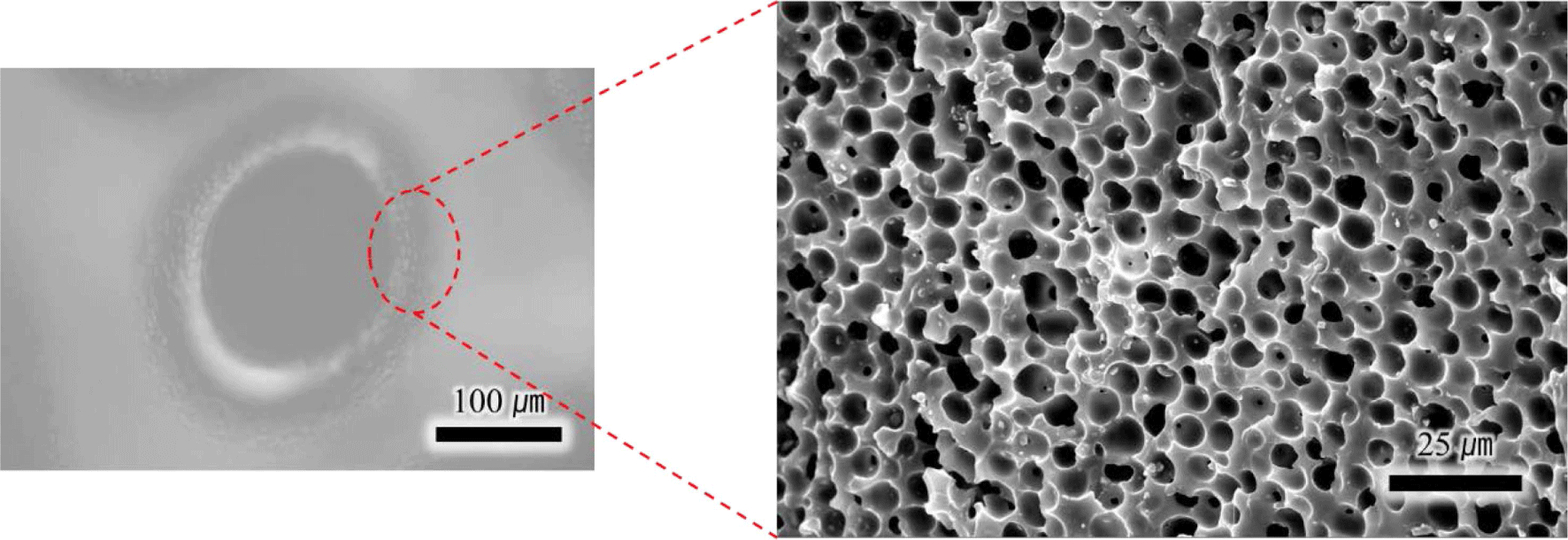
The difference between hydrocarbon-based PBA lies in the boiling point. Cyclohexane’s boiling point is closer to the curing temperature than that of n-hexane. Figure 8(a) and (b) illustrate that both the pore size and apparent density reach their minimum value at 0.5 phr (the dotted line represents the results of the neat foam). As previously discussed, when more than 1 phr was incorporated, the structure collapsed and cured without foaming, resulting in a product similar to rubber. Because hydrocarbon-based PBA has a low viscosity, it was not uniformly dispersed. And when an excessive amount was added, it appeared that they were combined with each other, forming large pores because of the excessive generation of bubbles. In Figure 8(a), the pore size appeared smaller when n-hexane was added compared to cyclohexane, and it was almost similar in size to the neat foam. When n-hexane was added, large pores formed at the bottom of foams. Thus, it seemed that almost all the n-hexane vaporized at the beginning of the foaming process and disappeared before the curing reaction could occur. As a result, the apparent density of the foam with added n-hexane (Figure 8(b)) was almost similar to that of the neat foam. On the other hand, due to the appropriate vaporization rate, the cyclohexane reduced the density by a maximum of 15%.
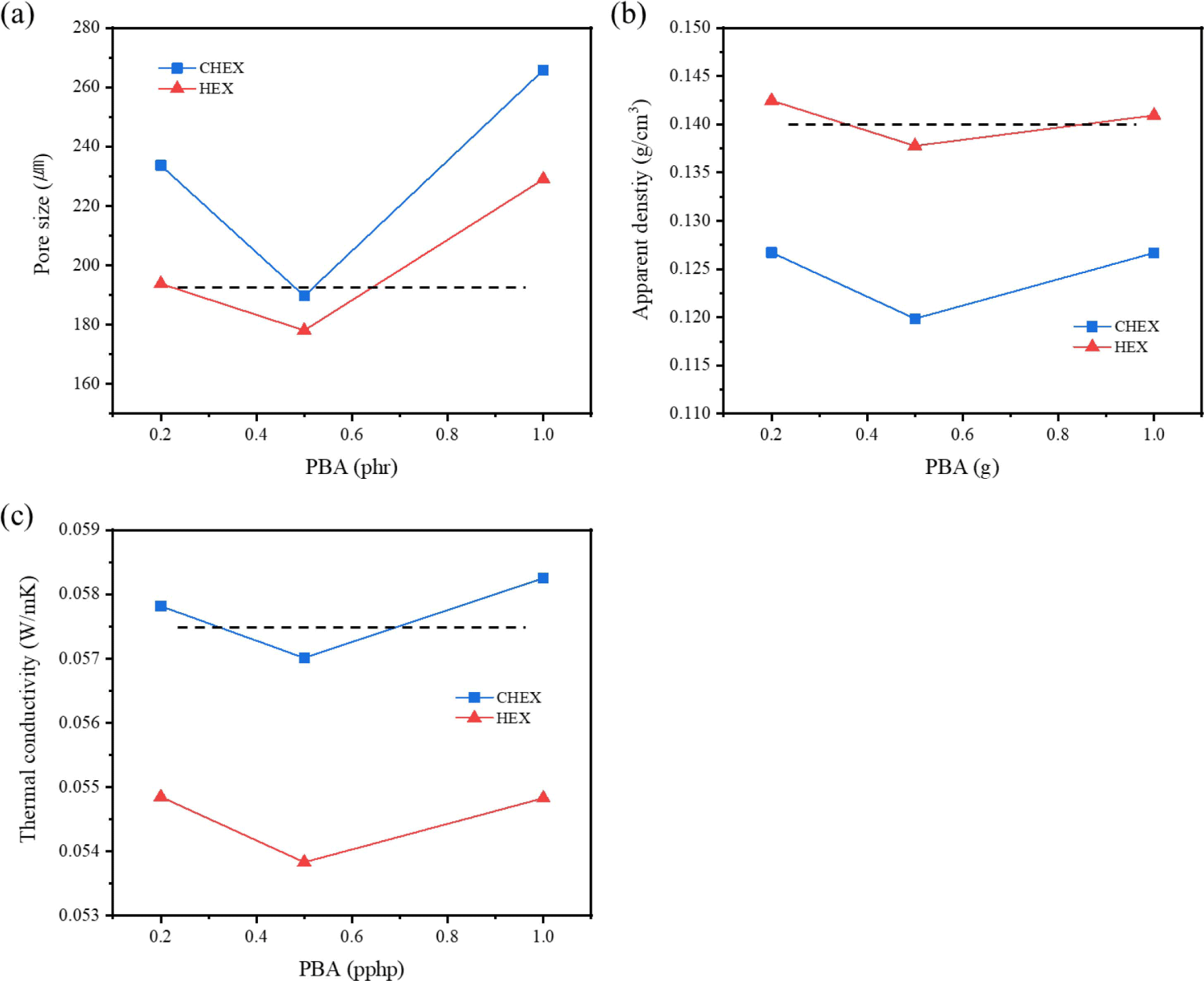
In Figure 8(c) representing the thermal conductivity, the addition of cyclohexane did not significantly change the that of the neat foam. Conversely, the addition of n-hexane resulted a more considerable impact on thermal conductivity. When n-hexane was added, the thermal conductivity decreased 57.5 to 53.8 mW/mK. It appears that the content of closed cell generated by hydrogen gas was increased with n-hexane and the addition of cyclohexane increased the number of types of gases involved in the foaming process to three (CO2, H2, and vaporized cyclohexane), and the larger pore size leads to increased thermal conductivity.
Figure 9 presented the thermal conductivity of hybrid foams incorporating various amounts of TEM. It is noted that TEM was added up to 3 phr since the increased viscosity interfered with the foaming process above 4 phr of TEM. It can be observed that with the addition of TEM, both pore size and apparent density decreased as the TEM quantity increases. When 3 phr of TEM was added, the apparent density decreased to 0.116 g/cm3 and the pore size also reduced to 127μm. The slight increase in viscosity facilitated a more even distribution of cell compared to the liquid state of hydrocarbon-based PBA and appears to be the reason for the stable generation of bubbles. On the other hand, the thermal conductivity appears higher than when hydrocarbon-based PBA was added. Typically in open cell foam, as the pore size decreases, the thermal conductivity decreases due to restricted air flow. However, when 1 to 2 phr of TEM was added, the thermal conductivity increased to more than 60 mW/mK, but decreased when 3 phr is added. This is presumably because at 1 to 2 phr, the pore size is similar to that of neat foam, but the thermal conductivity increases due to the high thermal conductivity of TEM itself. However, the addition of 3 phr TEM significantly reduces the pore size and effectively restricts air flow, resulting in the decrease in thermal conductivity.
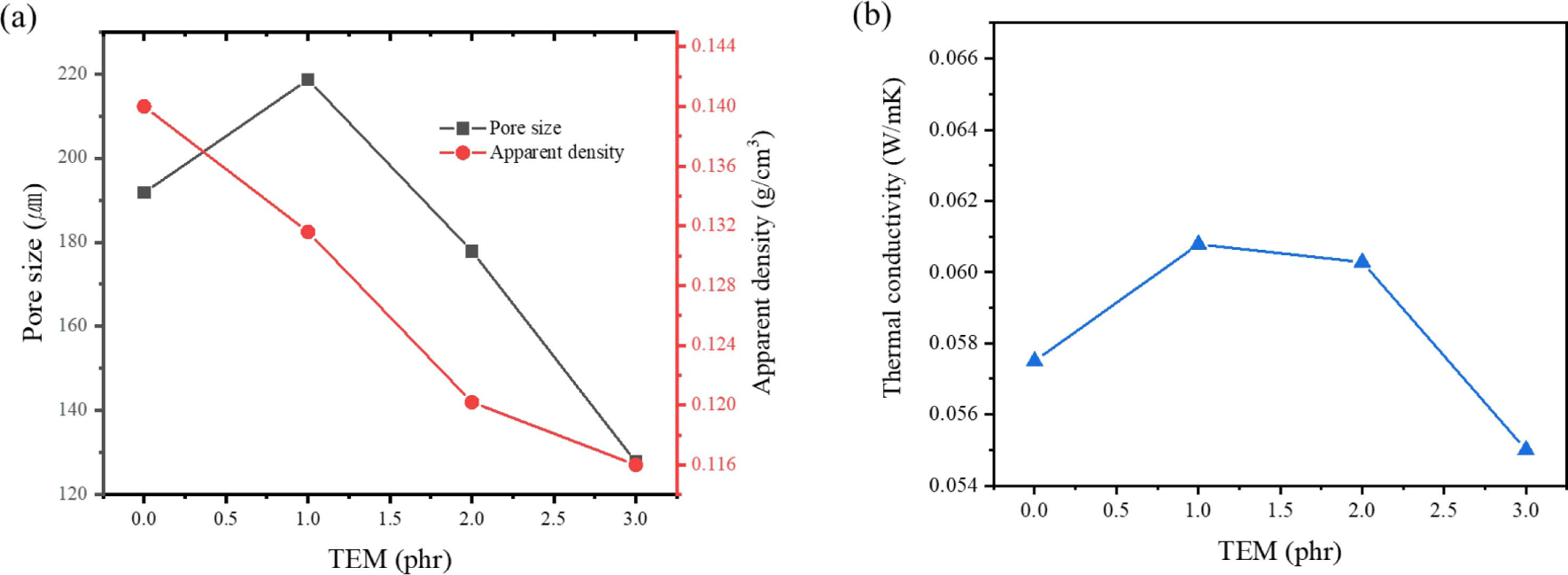
Conclusions
In summary, we demonstrated PU-PDMS hybrid foams with different types of PBA compared with neat hybrid foam. The hybrid foam was prepared with different contents of PBA. It appeared that most hydrocarbon-based PBA with relatively low boiling point such as n-hexane vaporized in the early stages of the foaming process, thus not greatly affecting the reduction in apparent density. However, cyclohexane with a boiling point similar to the curing temperature could participate in foaming process and reduced apparent density of the foam. Cyclohexane, while participating in the foaming process to reduce the density of the foam, created bubbles with CO2 and H2 gas. So, the excess bubbles combine to form larger pores. On the other hand, TEM leads to an increase in viscosity, which limits its addition to no more than 4 phr. However, its ability to reduce density and pore size according to content surpasses that of hydrocarbon-based PBA. The increase in the foaming height of the foam suggested that TEM expanded within the matrix of the foam. And with a minor increase in viscosity when added, the addition of microspheres resulted in a uniform distribution and bubble could be generated in a more stable state. However, in terms of thermal conductivity, no reduction effect was observed. The addition of cyclohexane led to an increase in thermal conductivity compared to the addition of n-hexane, due to the increase in pore size. Furthermore, the excess addition of TEM appeared to have increased thermal conductivity due to the high thermal conductivity intrinsic to the microsphere itself. Therefore, additives such as TEM can be applied more effectively than hydrocarbon-based PBA for reducing the density and pore size of PU-PDMS hybrid foam.