Introduction
Automotive tires are composed of ten or more layers, such as treads, sidewalls, composites, belt layers, and carcasses. Among them, the tread is the outermost layer of the tire, which is the most important layer because it contacts the ground directly. The tread is made of rubber that is resistant to breakage and impact, to protect the conductor and belt layers inside the tire, and wear-resistant rubber to increase tire life.
It is difficult to simultaneously improve the rolling resistance, wear resistance, and wetting property of the tire tread. Among these three properties, improvement of one physical property causes deterioration of another. Moreover, controlling these properties is very important for the manufacturing tire tread. The wear of the tire describes the phenomenon whereby the surface of the tread rubber contacting the ground is worn out because of the frictional force generated between the tread and the road surface. The wear of the tire considerably affects the life and braking performance of the tire. In addition, in terms of cost reduction, the tire tread should have excellent wear resistance.1
Owing to their good dispersion characteristics, carbon black fillers can facilitate the preparation of good-quality rubber compounds. However, the hysteresis of the rubber compounds increases, which is disadvantageous in terms of the fuel economy of the tire.2 In recent years, rubber compounds containing silica fillers have become increasingly popular because of reduced rolling resistance in tires and a significant improvement in the frictional force on wet and snow-covered roads. In particular, the silica content of ultra-high-performance tires and winter tires sold recently may exceed 100 phr. However, there are many difficulties associated with the preparation of compounded rubber using silica that exhibits satisfactory performance.3,4
Since silanol groups are present on the surface of the silica, hydrogen bonds can form between the hydroxyl groups on the silica surface; thus, the silica dispersibility of the rubber compounds decreases, causing aggregation of the silica particles. The phenomenon of silica aggregation adversely affects the properties of the rubber compound.5 Recently, many studies have been developed to improve the dispersibility of composites in various materials fields, and the wet masterbatch (WMB) process was developed to improve the silica dispersibility of rubber compounds.6 WMB rubber compounds are prepared by obtaining a solid rubber compound using a flocculant to solidify the sludge in which the filler-containing dispersion is mixed with the rubber latex. The use of the WMB process improves silica dispersibility and thus the mechanical properties of the resulting rubber compounds, in comparison to that achieved by mixing rubber and silica through the dry masterbatch (DMB) process. Typical rubber compounds manufactured by the WMB process have low rolling resistance and improved fatigue resistance.7
Previous studies have shown that the WMB process can load more than 100 phr of silica into the rubber compound; however, this reduces silica dispersion, which adversely affects the mechanical properties, abrasion resistance, and rolling resistance of the compound. While various silica dispersants are commercially available as additives for compounds in the DMB process, commercial silica dispersants suitable for the WMB process have not yet been explored.8-10 Recently, the development of silica dispersants applicable to WMB process has gained considerable attention. Some researchers have prepared dispersants using anionic polymers such as poly (acrylic acid), styrene-acrylic acid copolymers, and vinyl naphthalene-acrylic acid copolymers to enhance filler dispersion in WMB.11,12
Currently, researchers are focusing on the development of eco-friendly tire materials as a measure to reduce global warming; thus, rubber additives such as silica dispersants must be developed using eco-friendly materials.13,14 An example of relevant and eco-friendly material is cellulose, which is naturally available and has the chemical composition similar to that of starch. Previous studies have shown that the addition of cellulose to rubber compounds in the DMB process improved the environmental friendliness, biodegradability and processability, mechanical properties, and dynamic mechanical performance of the tire.15,16 Since cellulose is composed of carbon chains and hydroxyl groups, it exhibits both hydrophilic and hydrophobic functionalities. Because of these characteristics, research has been conducted using cellulose as a dispersant in various fields.17-19 However, the use of cellulose as a silica dispersant for tire manufacturing using the WMB process has not yet been investigated.
In this study, cellulose variants having large numbers of hydroxyl groups were investigated for their use as silica dispersants in the WMB process with the aim of preventing aggregation and improving the silica dispersibility. In the WMB process, rubber compounds were prepared using various cellulosic materials as silica dispersants. In order to assess the silica dispersibility, the processability, mechanical properties, and wear resistance of the rubber compounds were evaluated.
Experimental
Cellulose (Powder 20 μm, Sigma Aldrich, United States), 2-hydroxyethyl cellulose (Powder, Sigma Aldrich, United States), and cellulose acetate (Powder, Sigma Aldrich, United States) were used without further purification. ESBR 1712 (KUMHO PETROCHEMICAL, South Korea) was the rubber latex used for the WMB process, and a 2% w/w CaCl2 aqueous solution was used as a coagulant. Modified silica NK153 (MIRAESI, South Korea) was used as a reinforcing agent for the compound, whose surface was hydrophobically modified with 10% w/w bis [3-(triethoxysilyl) propyl] tetrasulfide (TESPT). Various compounding processing additives such as zinc oxide (ZnO), stearic acid (S/A), and N-(1,3-dimethyl-butyl)-N'-phenyl-p-phenylenediamine (6PPD, Sigma Aldrich, United States) were used. In the final compounding step, sulfur (SAMCHUN, South Korea) was used as a crosslinking agent. N-cyclohexyl-2-benzothiazole sulfonamide (CBS, Tokyo Chemical Industry, Japan) and diphenyl guanidine (DPG, Sigma Aldrich, United States) were used as crosslinking accelerators.
The silica–SBR compounds were prepared using three processes: WMB, silica master match (SMB), and final master batch (FMB). In the WMB process, the emulsion styrene butadiene rubber (ESBR) was added to silica sludge. After stirring, the cellulose-based dispersants were added, as solutions dissolved in water, and the coagulant was added while stirring to aggregate the ESBR and load the silica. In the SMB process, ZnO, S/A, and 6PPD were added as the primary mixing agents. The FMB process is a secondary mixing process that prepares the silica–SBR compounds before vulcanization by adding sulfur for vulcanization as well as DPG and CBS as vulcanization accelerators.
Figure 1 illustrates the WMB, SMB and FMB processes for the preparation of silica–SBR compounds. TESPT-modified silica (2% w/w) was added to distilled water and stirred at 60°C to prepare a silica slurry. After mixing it with the ESBR, also heated to 60°C, the mixture was stirred for 10 min. A solution prepared by dissolving the cellulose-based dispersants in distilled water was then added to the silica and ESBR slurry. After 15 min the mixed slurry was coagulated with CaCl2, washed once, and dried at 60°C for 24 h.
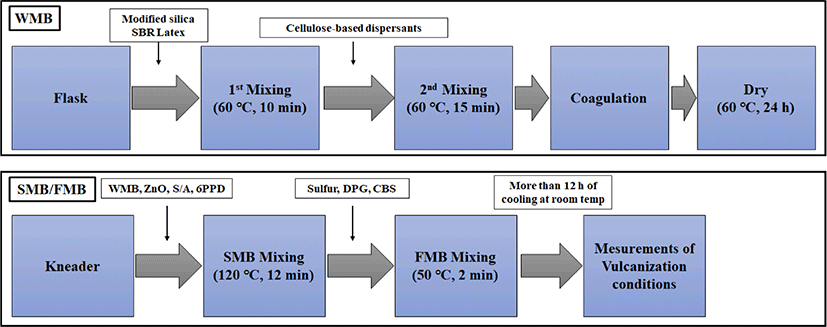
The SMB was produced using dried WMB compounds. The dried WMB compounds were mixed with ZnO, S/A, and 6PPD for 12 min at 120°C. The FMB was prepared using the SMB compounds, CBS, DPG, and sulfur. They were mixed for 2 min at 50°C using a two-roll mill for the preparation of the final silica–SBR compounds. The detailed formulations of silica-SBR compounds are shown in Table 1.
The cure characteristics and silica dispersibility of four silica–SBR compounds made according to Table 2 were measured by MDR. The MDR results confirmed that silica dispersibility was improved when cellulose was added for all three types of cellulose-based dispersants. Next, silica–SBR compounds were prepared with cellulose applied at 2, 4, and 6 phr levels, and the content of cellulose was confirmed.
Unit | WN | WC2 | WHC2 | WCA2 | |
---|---|---|---|---|---|
t10 | min:ss | 03:15 | 03:44 | 03:54 | 03:45 |
t90 | min:ss | 15:51 | 15:33 | 15:16 | 14:41 |
Tmin | N-m | 0.561 | 0.545 | 0.583 | 0.554 |
Tmax | N-m | 2.011 | 1.885 | 1.874 | 1.799 |
Tmax-Tmin | N-m | 1.450 | 1.340 | 1.291 | 1.245 |
The torque and cure time of the silica–SBR compounds in the FMB stage were measured with a moving die rheometer (MDR, MDR2020, MYUNGJI TECH) at 160 ± 1°C for 30 min. The optimum cure time was determined using an MDR based on ISO 6502. This test measures the minimum torque value (Tmin), maximum torque value (Tmax), scorch time (t10), and optimum cure time (t90).
The Mooney viscosity (MV, MV2020, MYUNGJI TECH) of the silica–SBR compounds in the FMB stage provides a measure of the processability of the unvulcanized rubber. In ML(1+4), the “M” means Mooney, the “L” means plate size, the “1” means 1 min preheating and the “4” corresponds to the measured value after 4 min of rotor operation. Generally, when the value of ML(1+4) is low, the fluidity is considered to be good, and the processability to be excellent. The measurement of the MV (ML(1+4)@100°C) was performed using a Mooney viscometer as per ISO 289-1.
The modulus, tensile strength, and elongation of the silica–SBR compounds were measured according to the ASTM D412 standard. A universal testing machine (H5KT-0635, Tinius Olsen) was used to measure these parameters. The wear resistance of the silica–SBR compounds was measured using a DIN wear tester (AT-2020-D, MYUNGJI TECH) in accordance with the ASTM D 5963 standard.
Results and Discussion
Figure 2 illustrates the dispersing mechanism of cellulose in the WMB process. In the case of modified silica, the TESPT-modified portion is hydrophobic, but the unmodified portion has hydroxyl groups. Silica aggregation occurs due to hydrogen bonding between the hydroxyl groups, which reduces the silica dispersibility of the silica–SBR compounds. Cellulose adheres to hydroxyl groups present on the surface of silica via hydrogen bonding to prevent aggregation of silica, improving the silica dispersibility in the WMB process.
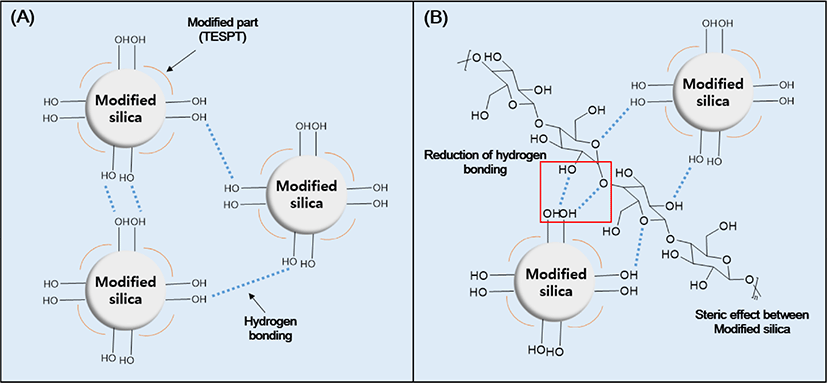
The cure properties of the four rubber compounds prepared according to the formulations of Table 1 were confirmed by MDR. The MDR results of silica-SBR compounds with and without cellulose-based dispersants are shown in Figure 3 and Table 2. The t10 indicates the time when the vulcanization of the silica–SBR compound is 10%, also known as the scorch time of the rubber. A longer t10 time correlates to better processability of the silica–SBR compound. Our results show that the t10 increased for both samples with cellulose-based dispersants. Specifically, for WHC, the t10 value increased by 40 s when compared to that of the corresponding material without a dispersant (WN). The t90 parameter represents the cure time when rubber vulcanization is 90% complete, and is called the optimum vulcanization time. In this study, the t90 values decreased for all three silica–SBR compounds prepared with cellulose-based dispersants. The Tmin indicates the minimum torque of silica–SBR compounds and tends to decrease as the silica dispersibility improves. It has been shown that dispersants other than cellulose increased Tmin when compared to rubber materials prepared without dispersants, which has an adverse effect on silica dispersibility. The Tmax represents the maximum torque of the silica–SBR compound and tends to increase as the crosslinking density increases. In the case of cellulose-based dispersants, it was found that the Tmax decreased, which resulted in a decrease in the crosslinking density of the rubber. Hydrogen bonding between the CBS cure accelerator and cellulose may have led to a loss of CBS activity. The Tmax-Tmin represents the crosslinking density of the rubber compound, which is similar to the above-mentioned trend, and shows that the crosslinking density decreased when the cellulose-based dispersants were used. MDR results showed the lowest Tmin and the highest crosslinking density for silica-rubber compounds prepared with cellulose (WC) among the three types cellulose-based dispersants used. Therefore, we chose to examine cellulose as a dispersant for the remainder of this study.
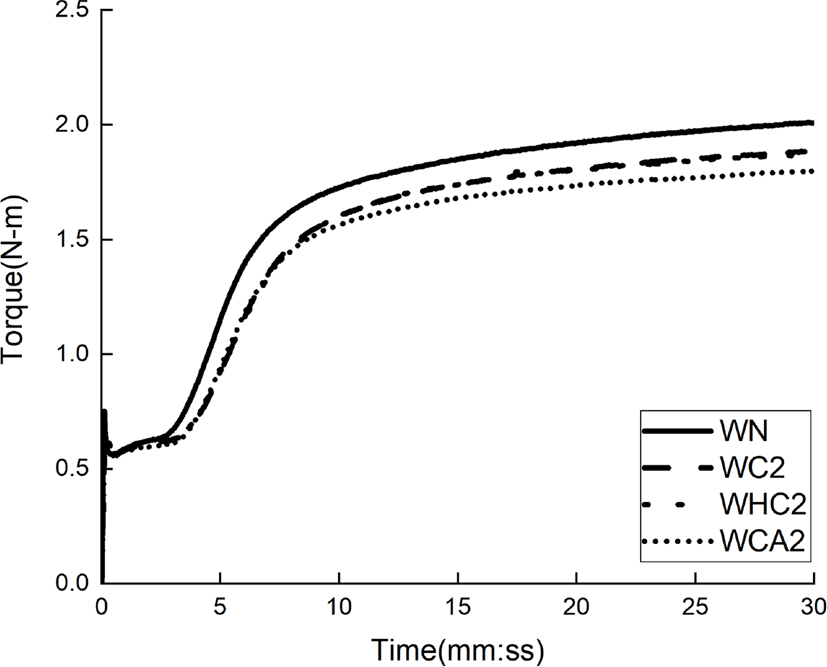
The cure characteristics of compounds according to cellulose content are shown in Figure 4 and listed in Table 3. As the cellulose content increases, the t90 decreases. In the case of WC06, the t90 was reduced by 1 min or more when compared with WN, which is due to the improved silica dispersibility. The Tmin, values measured, decreased with increasing cellulose content, with WC06 being reduced by up to 7% when compared with WN. This shows that cellulose interferes with the aggregation of silica through hydrogen bonding with the silica. Tmax and Tmax-Tmin values of the WC series using cellulose dispersants decreased compared to those of WN, which was due to the lower activity of CBS caused by a higher degree of hydrogen bonding between CBS and cellulose.
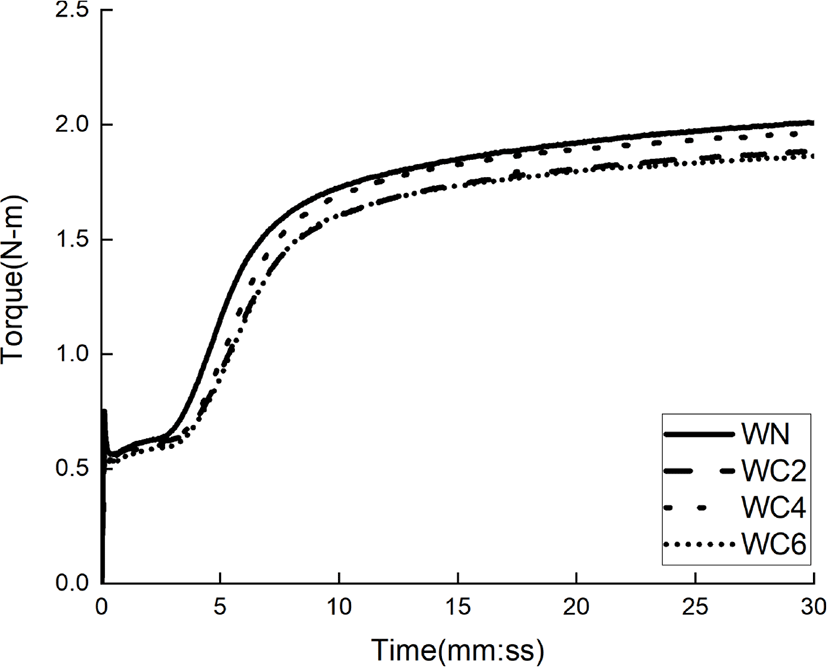
Unit | WN | WC2 | WC4 | WC6 | |
---|---|---|---|---|---|
t10 | min:ss | 03:15 | 03:44 | 03:37 | 03:41 |
t90 | min:ss | 15:51 | 15:33 | 14:49 | 14:46 |
T min | N-m | 0.561 | 0.545 | 0.534 | 0.525 |
Tmax | N-m | 2.011 | 1.885 | 1.967 | 1.865 |
Tmax-Tmin | N-m | 1.450 | 1.340 | 1.433 | 1.340 |
Figure 5 and Table 4 provide comparisons of the MV values for the silica–SBR compounds in the FMB stage. ML(1+4) is the MV index, indicating the processability for the rubber material. A low MV value implies high processability of the silica–rubber compound, indicating good silica dispersion.20,21 In case of the compounds of WHC and WCA, the MV index was impossible to measure, which imply that 2-hydroxyethyl cellulose and cellulose acetate are not suitable silica dispersants. As the cellulose content increased, the MV values of the silica–rubber compounds decreased. Similar to the Tmin results of MDR, ML(1+4) decreased with increasing cellulose content by up to 7% for WC06 compared to WN. Cellulose has been shown to improve processability by preventing the agglomeration of silica and consequently improving the silica dispersibility of the silica–SBR compounds.
Unit | WN | WC2 | WHC2 | WCA2 | WC4 | WC6 | |
---|---|---|---|---|---|---|---|
Initial | Torque | 192.8 | 178.6 | over | over | 183.1 | 172.1 |
ML(1+4) | Torque | 93.6 | 92.9 | over | over | 90.5 | 87.2 |
The mechanical properties of the silica–SBR compounds are listed in Table 5. The M100% and M300% represent the moduli of the silica–SBR compounds at 100% and 300% elongation, respectively. All mechanical properties of the WC compounds were higher than those of WHC and WCA compounds. As the content of cellulose increased from 0 to 4 phr of cellulose, the M100% and M300% values increased, and then decreased for WC06, which contains 6 phr of silica. This is because cellulose improves the silica dispersion in the silica–SBR compounds, thereby improving the tensile strength of the rubber, until the 4 phr mark. When the content of cellulose is higher, for example at 6 phr, the tensile strength of WC compounds is lowered because the remaining cellulose reduces the crosslinking density of the rubber by weakening the activity of the CBS vulcanization.
Figure 6 graphically depicts the wear resistance of the silica–SBR compounds. The WN compound shows the highest weight loss among the compounds. Comparing the wear resistance of WHC and WCA compounds, those of WC series show the higher wear resistance, due to the poor silica dispersibility. Particularly, the WC04 compound had the best wear resistance with a weight loss decrease of up to 29% when compared to the rubber compound without cellulose (WN). This is believed to be due to improved silica dispersibility, resulting in smaller silica particles with increased surface area. Notably, the wear resistance deteriorated in the case of WC06. This is once again due to remaining cellulose in the compound resulting in lowered crosslinking density. This is also due to the deterioration of the activity of the vulcanization accelerator through hydrogen bonding between the cellulose and the vulcanization accelerator, similar to the tensile strength.
Conclusions
In this study, silica-SBR compounds were prepared by adding cellulose-based dispersants to the WMB process and subsequently evaluated. We confirmed that cellulose was the best dispersant through experiments comparing three cellulose-based dispersants, i.e., cellulose, 2-hydroxyethyl cellulose, and cellulose acetate. Vulcanization properties, processability, mechanical properties, and wear resistance of the cellulose containing compounds (WC) were measured. The cure rate of the WC series was higher than that of rubber compounds without cellulose. As the content of cellulose increased, the ML(1+4) values decreased, indicating that the silica dispersibility of the compounds had increased, thereby improving the processability of the silica-SBR compound. The mechanical properties of WC02 and WC04, with 2 and 4 phr of cellulose, respectively, were improved in terms of tensile strength. However, the mechanical properties of the WC compound with 6 phr were reduced. This seems to be a result of deactivation of CBS through hydrogen bonding between cellulose and CBS. The wear resistance of the WC compounds was enhanced with increasing cellulose content, but decreased again when cellulose content approached 6 phr. This is due to the presence of a large amount of cellulose having a low modulus, which is similar to the phenomenon observed for the tensile strength.