Introduction
Organic dyes can cause vomiting, pain, hemorrhage, ulceration of skin and mucous membranes, and dermatitis.1 In addition, the presence of large amounts of organic dyes in wastewater can cause the toxic environment to aquatic organisms, inhibit the penetration of sunlight, and reduce the rate of photosynthesis.2 Therefore, the removal of organic dyes from wastewater is imperative for preserving the natural environment. MO is widely used as a coloring agent for the detection of hydrogen gas in the food, leather, and pharmaceutical industries.3,4 Various physical (coagulation, reverse osmosis, membrane filtration), chemical (reduction, oxidation, ion exchange, complexometric), and biological (aerobic and anaerobic) methods are used for removing MO from wastewater.5 Among these methods, photocatalytic degradation is the most efficient approach for removing dyes and other pollutants from wastewater. Photocatalytic degradation converts organic dyes completely to H2O, CO2, and less or nontoxic compounds without causing secondary pollution.6
Advanced oxidation processes are usually used for the degradation of organic dyes in wastewater.7-10 These mechanisms involve the formation of hydroxyl (·OH) and superoxide (·O2−) radicals.11 Semiconductor metal oxides such as ZnO can act as catalysts for the degradation of organic dyes in wastewater.2
Zinc oxide is an n-type metal oxide semiconductor with a wide band gap of 3.37 eV and a large exciting binding energy of 60 meV at room temperature.12 Because of these properties, ZnO is used as antibacterial agents and in solar cells, light emitting diodes, nano lasers, piezoelectric devices, ultraviolet (UV) shielding materials, and gas sensors.7-9,13-15
However, in ZnO, the recombination rate of photo-generated electron-hole pairs is usually faster than the surface redox reaction, which severely limits its practical use, especially in the photocatalytic degradation of organic compounds.2 Therefore, various studies have been carried out to suppress the electron-hole pair recombination in ZnO in order to improve the photocatalytic efficiency. The commonly used approaches for suppressing the electron-hole pair recombination of ZnO include combining ZnO with other semiconductors8-11,16,17 or carbon-based materials18-26 and doping with metals27-34 and nonmetals.35-38
When combined with carbon nanotubes39,40 and carbon nanofibers,41,42 ZnO shows excellent photocatalytic activity. In particular, carbon-based nanomaterials act as an electron acceptors. It acts as an electron transport materials in the photocatalytic degradation of organic dyes and facilitates the migration of photo-generated electrons and suppressing the charge recombination, thus enhances the lifetime of electron-hole pairs and improves the photocatalytic efficiency of the composite.43
(C60) Fullerene nanowhiskers are composed of single-crystal (C60) fullerene, a typical carbon nanomaterial, and thin needle-like fibers with a length less than 100 μm.44 These nanowhiskers are used in many applications such as in chemical sensors, solar cells, photosensors, and field-effect transistors.45-50 The nanowhiskers are prepared by the liquid–liquid interfacial precipitation (LLIP) method.46
(C60) Fullerene nanowhiskers exhibit unique properties such as high charge carrier mobility,51 optical transmittance,45 and higher Young’s modulus than that of the pristine (C60) fullerene crystal.52 Owing to these unique properties, (C60) fullerene nanowhiskers act as excellent electronic acceptors and electron transport materials in hybrid nanocomposites.
Various efforts have been made to develop hybrid nanocomposites of semiconductor materials and (C60) fullerene nanowhiskers with excellent nanocatalytic performance.53,54
In this study, we synthesized a zinc oxide nanoparticle-(C60) fullerene nanowhisker nanocomposite from zinc oxide nanoparticles using the LLIP method.
The zinc oxide nanoparticle and zinc oxide nanoparticle-(C60) fullerene nanowhisker composite were used to degrade MO under UV (at 254 and 365 nm) and ultrasonic irradiation.
Experimental
(C60) Fullerene was obtained from Tokyo Chemical Industry Co. Ltd. MO and hydrogen peroxide (H2O2, 30%, w/w) were purchased from Daejung Chemicals. Zinc nitrate hexahydrate (Zn(NO3)2∙6H2O), sodium hydroxide (NaOH), toluene (C7H8), ethanol (C2H5OH), and 2-propanol (C3H8O) were supplied by Samchun Chemicals.
An electric furnace (Ajeon Heating Industry Co., Ltd.) was used to heat the samples. The photocatalytic degradation of MO was confirmed using ultraviolet-visible (UV-Vis) spectroscopy (Shimazu UV-1601 PC) under ultraviolet and ultrasonic radiation condition. An ultraviolet lamp(8 W, 254 nm/365 nm, 77202 Marne La Vallee-Cedex 1, France) was used for irradiation of UV light. The ultrasonic radiation of 20 kHz frequency was generated using UGI1200 (Hanil Ultrasonic Co., Ltd.), equipped with a horn-type tip of 13 nm OD. The crystal structures of the samples were analyzed using X-ray diffraction (XRD) (Bruker, D8 Advance) at 40 kV and 40 mA. Raman spectroscopy (BWTEK, BWS465-532S) was used to analyze the lattice vibrations of the sample. Scanning electron microscopy (SEM) (JEOL, Ltd., JSM-6510) measurements were carried out at an accelerating voltage of 10 kV to examine the surface morphologies of the samples.
Zinc oxide nanoparticle was prepared by mixing 1 M zinc nitrate hexahydrate (Zn(NO3)2·6H2O) and 10 M of sodium hydroxide (NaOH) with 20 mL of distilled water followed by stirring for 30 min. The resulting solution was subjected to ultrasonic irradiation for 45 min. The mixture was then washed with distilled water and ethanol by centrifugation and placed in an electric furnace at 200°C for 1 h under the flow of Ar gas to obtain zinc oxide nanoparticles.
The zinc oxide nanoparticle-(C60) fullerene nanowhisker composite was prepared using the LLIP method by blending the zinc oxide nanoparticle solution (~0.2 mg/mL), C60-saturated toluene (~14 mg/mL), and isopropyl alcohol at a volume ratio of 1:14:75. The resulting solution was kept at 5℃ for 20 h.
The catalytic activities of the zinc oxide nanoparticle and zinc oxide nanoparticle-(C60) fullerene nanowhisker composite for the degradation of MO were evaluated. Stock MO solutions were synthesized using 2 mM of MO powder. The zinc oxide nanoparticle and zinc oxide nanoparticle-(C60) fullerene nanowhisker composite (0.1 g/L) were used for the photocatalytic degradation of a 4.2 × 10−2 mM MO solution containing H2O2. The pH of the MO solution was maintained at 5.7, H2O2 was used as an oxidizing reagent. The solution was stirred for 30 min in the absence of light to attain an adsorption-desorption equilibrium between the MO molecules and the catalysts.
The catalytic degradation process was monitored using UV-Vis spectroscopy. The catalytic degradation of MO over the zinc oxide nanoparticle and zinc oxide nanoparticle-(C60) fullerene nanowhisker composite was carried out under UV (at 254 and 365 nm) and ultrasonic irradiation.
Results and Discussion
The crystal structures and crystallite sizes of the zinc oxide nanoparticle and zinc oxide nanoparticle-(C60) fullerene nanowhisker composite were examined using powder XRD. The XRD patterns of the zinc oxide and zinc oxide nanoparticle-(C60) fullerene nanowhisker composite are shown in Figure 1. The composite showed peaks at 2θ = 10.74°, 17.71°, 20.69°, 28.02°, 30.79°, and 32.75° corresponding to the (111), (220), (222), (420), (422), and (333) planes of (C60) fullerene nanowhisker, respectively. On the other hand, the peaks at 2θ = 31.77°, 34.42°, 36.26°, 47.54°, 56.61°, 62.86°, 67.39°, and 69.10° correspond to the (100), (002), (101), (102), (210), (103), (212), and (201) planes of zinc oxide nanoparticle, respectively (JCPDS card No.36-1451).55 The XRD results indicated the successful preparation of the zinc oxide nanoparticle and zinc oxide nanoparticle-(C60) fullerene nanowhisker composite. The crystallite sizes of the synthesized zinc oxide nanoparticle and zinc oxide nanoparticle-(C60) fullerene nanowhisker composite were calculated using the Scherrer formula as follows:
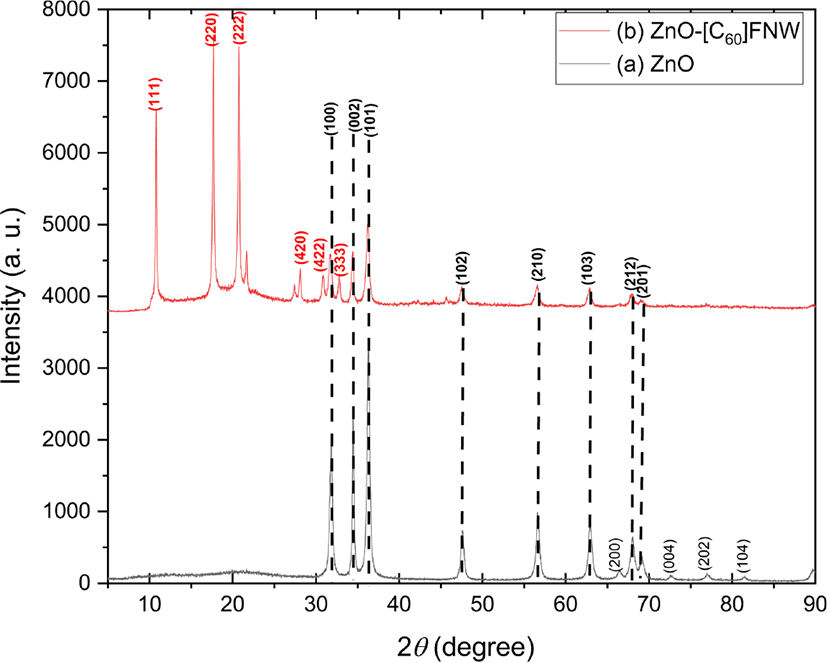
where D is the crystallite size, λ is the wavelength of Cu-Kα radiation (λ = 0.154 nm), k is the Scherrer constant (taken as 0.94), 2θ is the angle between the incident and scattered X-rays, and β is the full width at half maximum. The average crystallite size of the zinc oxide nanoparticle was found to be approximately 21.04 nm (Table 1).
Figure 2 shows the morphologies of the zinc oxide nano-particle and zinc oxide nanoparticle-(C60) fullerene nanowhisker composite photographed using SEM. The image of SEM revealed that most of the synthesized zinc oxide nanoparticle structure showed almost snow flower-like morphology in Figure 2(a). In the zinc nanoparticle-(C60) fullerene nanowhisker composite, with snow flower-like structure and agglomerated zinc oxide nanoparticle were observed on the (C60) fullerene nanowhisker with a needle-like structure in Figure 2(b).
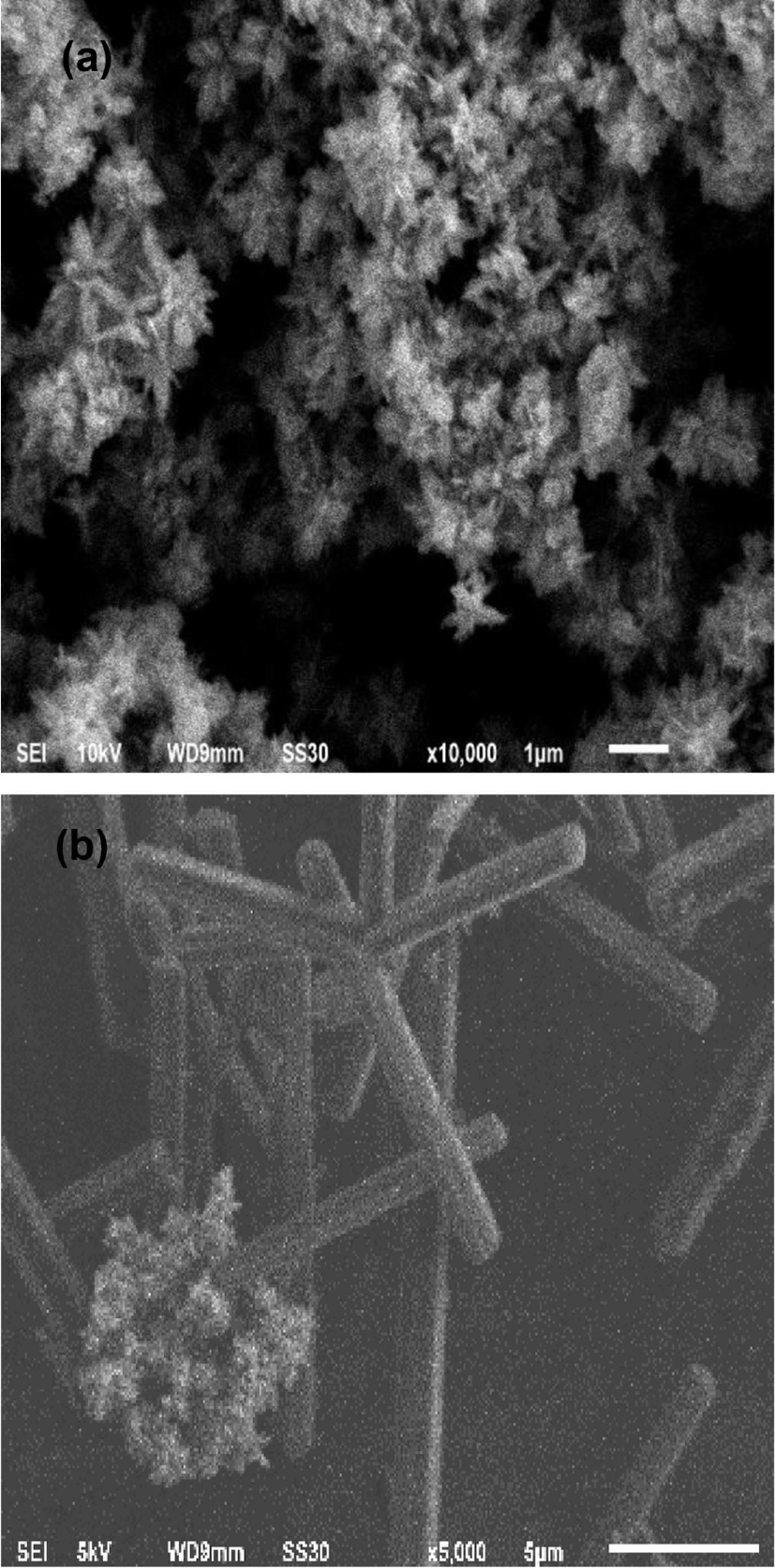
The vibrational modes of the zinc oxide nanoparticle and zinc oxide nanoparticle-(C60) fullerene nanowhisker composite were observed using Raman spectroscopy, as shown in Figure 3.
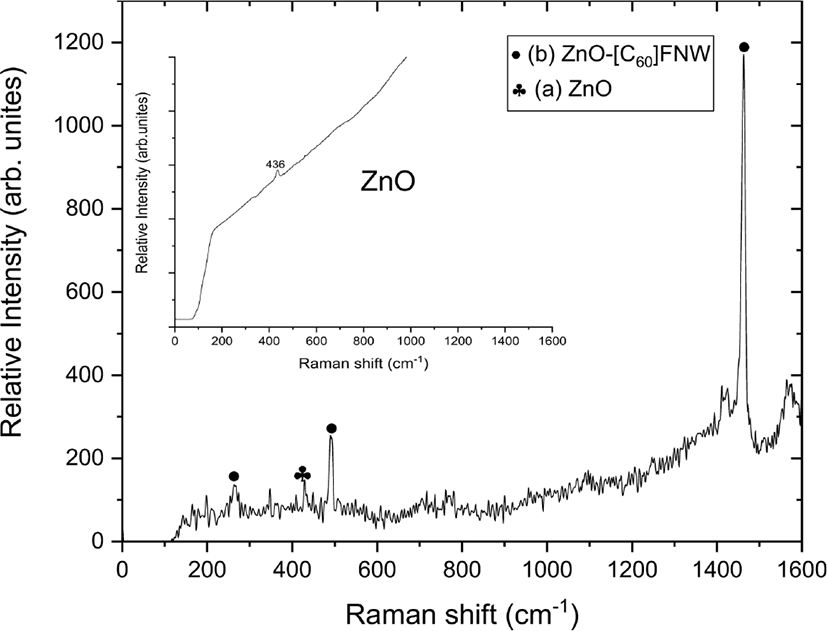
The zinc oxide nanoparticle-(C60) fullerene nanowhisker composite showed Hg(1), Ag(1), and Ag(2) Raman shifts at 263, 490, and 1460 cm−1, respectively because of the presence of (C60) fullerene nanowhisker. A peak corresponding to the E2h mode of the zinc oxide nanoparticle was observed at 436 cm−1.56 The peak shown at 430 cm−1 can be seen as the presence of zinc oxide nanoparticle because blue shift is generated by the (C60) fullerene nanowhisker.
The catalytic degradation rate of MO shows in the presence of zinc oxide nanoparticle under various conditions such as UV and ultrasonic irradiation in Figure 4. The MO degradation rate under various conditions decreased in the following order: 254 nm UV irradiation > 365 nm UV irradiation > ultrasonic irradiation.
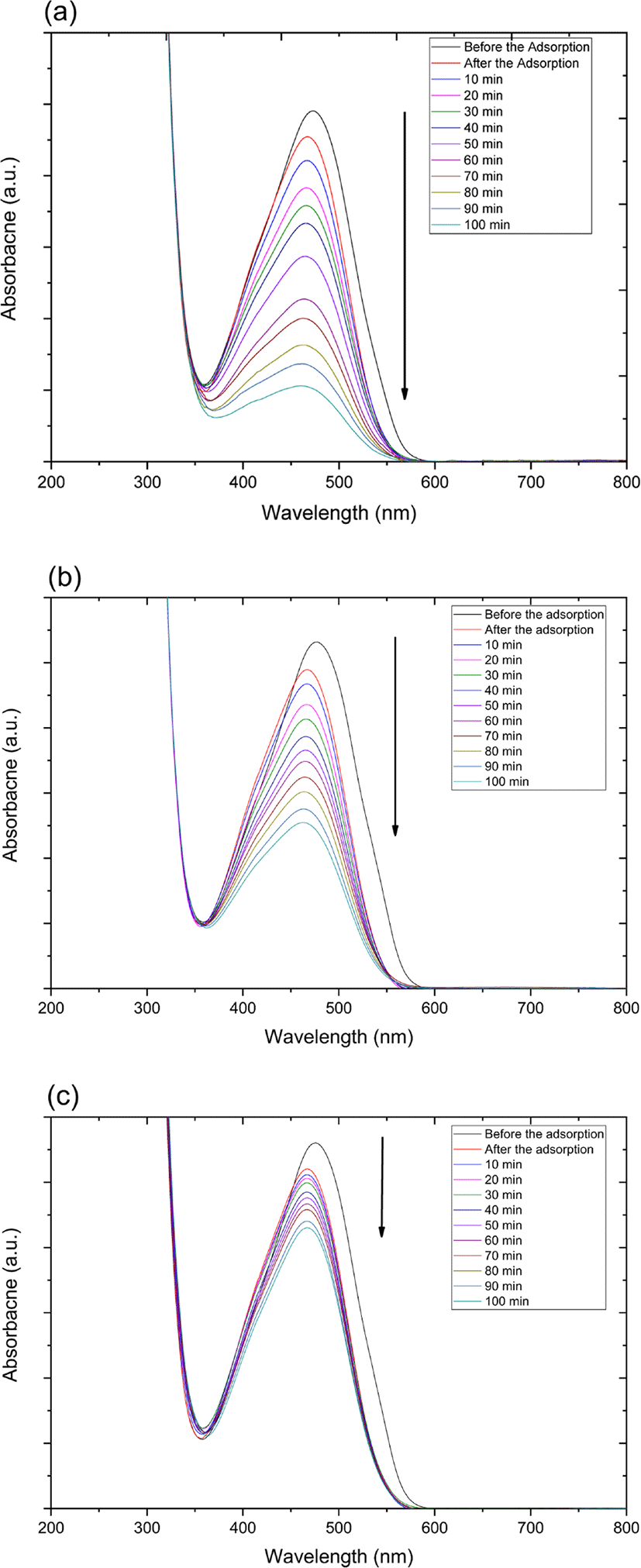
Also, catalytic degradation rate of MO using on zinc oxide nanoparticle-(C60) fullerene nanowhisker composite exhibits in Figure 5. The tendency of MO degradation rate under various conditions in Figure 5 decreased similarly to zinc oxide nanoparticle catalyst in the following order: 254 nm UV irradiation > 365 nm UV irradiation > ultrasonic irradiation.
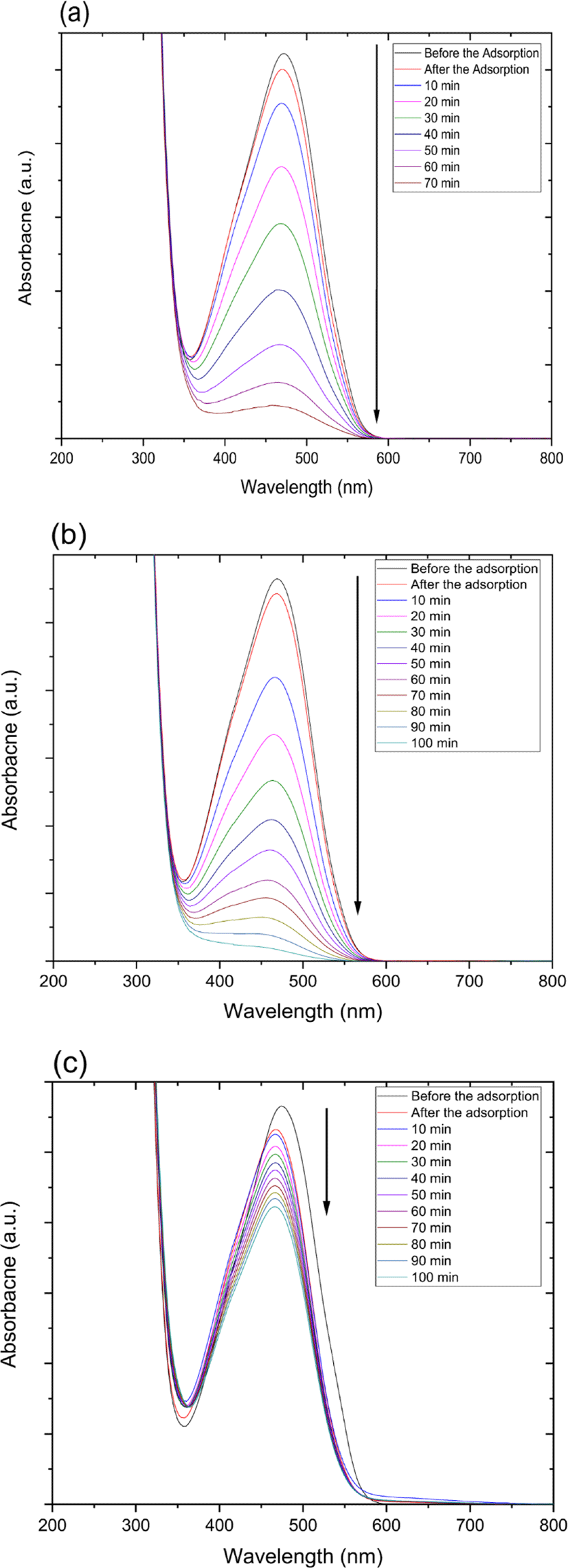
Figure 4 and 5 show the UV-Vis spectra for the catalytic degradation of MO over the zinc oxide nanoparticle and zinc oxide nanoparticle-(C60) fullerene nanowhisker composite as catalysts. As can be observed from Figure 6, the R2 values (coefficient of determination) for the pseudo first-order reaction kinetics were 0.997, 0.995, and 0.989. The catalytic degradation of MO was carried out under UV light (at 254 and 365 nm) and ultrasonic irradiation. The equation for the first-order reaction kinetics is as follows (Figure 6):
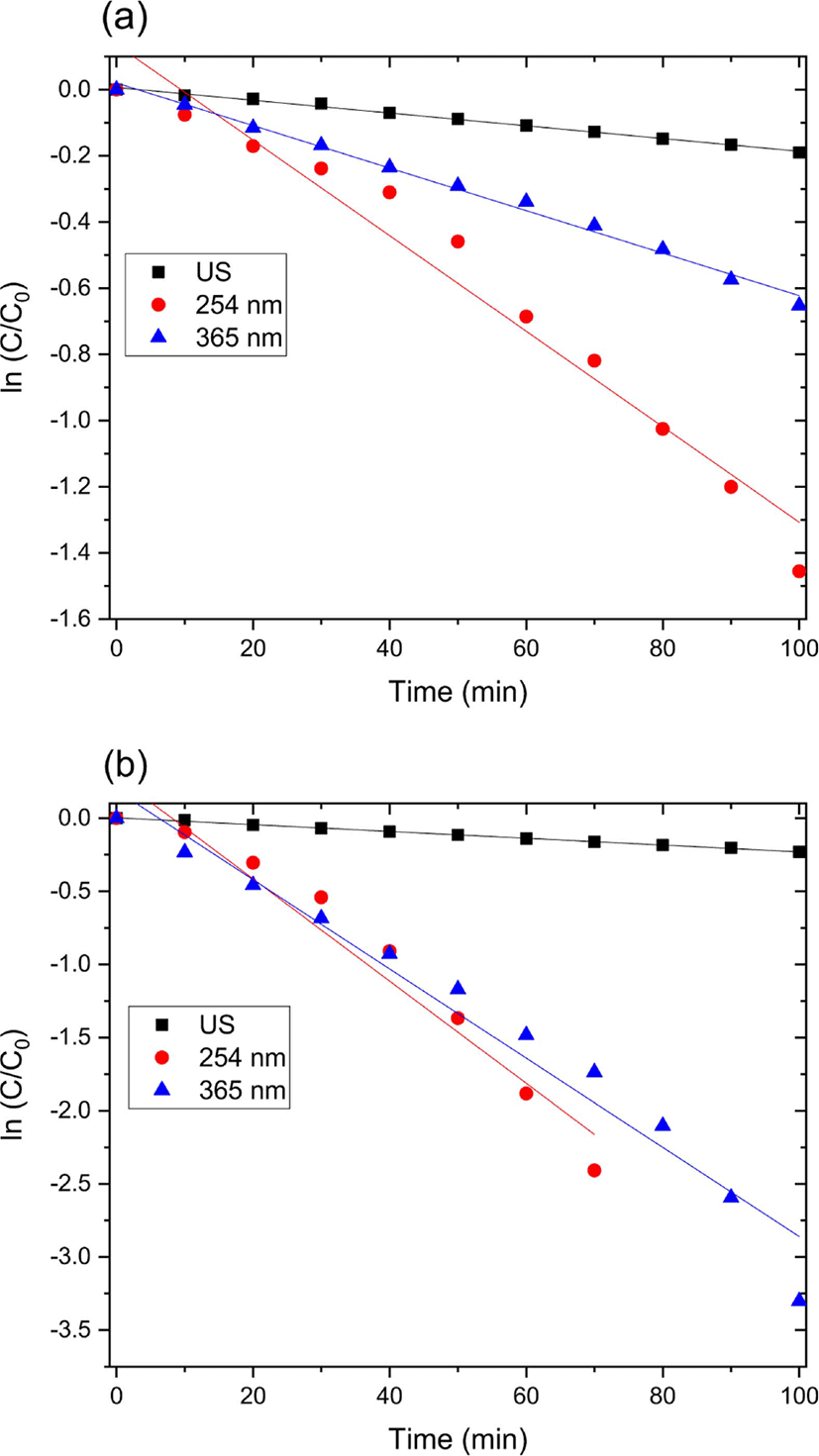
where C is the initial azo dye concentration and k1 is the first-order rate constant. The linear behavior of the curves indicates that the catalytic degradation of MO over the catalysts followed pseudo first-order kinetics.57
Conclusions
A zinc oxide nanoparticle-(C60) fullerene nanowhisker composite was prepared via the LLIP method using a zinc oxide nanoparticle solution and saturated (C60) fullerene in toluene and isopropyl alcohol. The hybrid nanocomposite was characterized using XRD, SEM, and Raman spectroscopy. The zinc oxide nanoparticle and zinc oxide nanoparticle-(C60) fullerene nanowhisker composite were used for the catalytic degradation of MO under UV (254 and 365 nm) and ultrasonic irradiation. The ZnO nanoparticle-(C60) fullerene nanowhisker composite showed better catalytic activity than the zinc oxide nanoparticle. The (C60) fullerene nanowhiskers in the composite acted as electron acceptors and electron transfer materials during the catalytic degradation of MO, thereby improved the efficiency of the composite. The MO degradation rate of the composite under various conditions decreased in the following order: 254 nm UV irradiation > 365 nm UV irradiation > ultrasonic irradiation. Overall, the zinc oxide nanoparticle-(C60) fullerene nanowhisker composite showed better catalytic degradation of MO than the ZnO nanoparticles. In addition, the kinetics of the catalytic degradation of MO over the hybrid catalyst followed the pseudo-first-order reaction rate law.